New 3D-Printing Process Uses Light to Make Complete Glass Microstructures
New 3D-Printing Process Uses Light to Make Complete Glass Microstructures
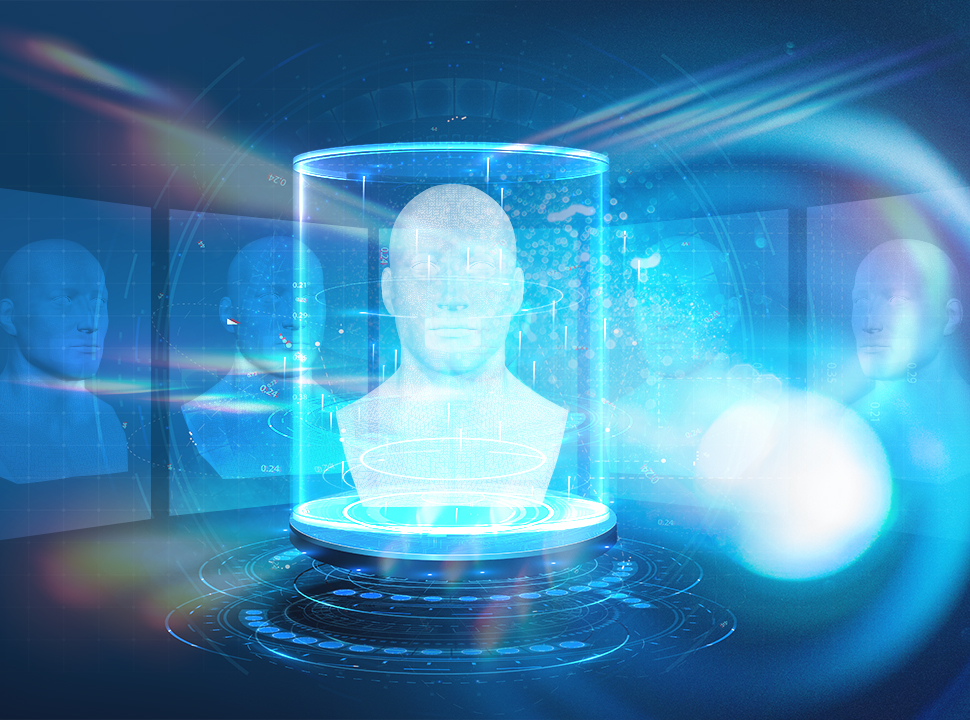
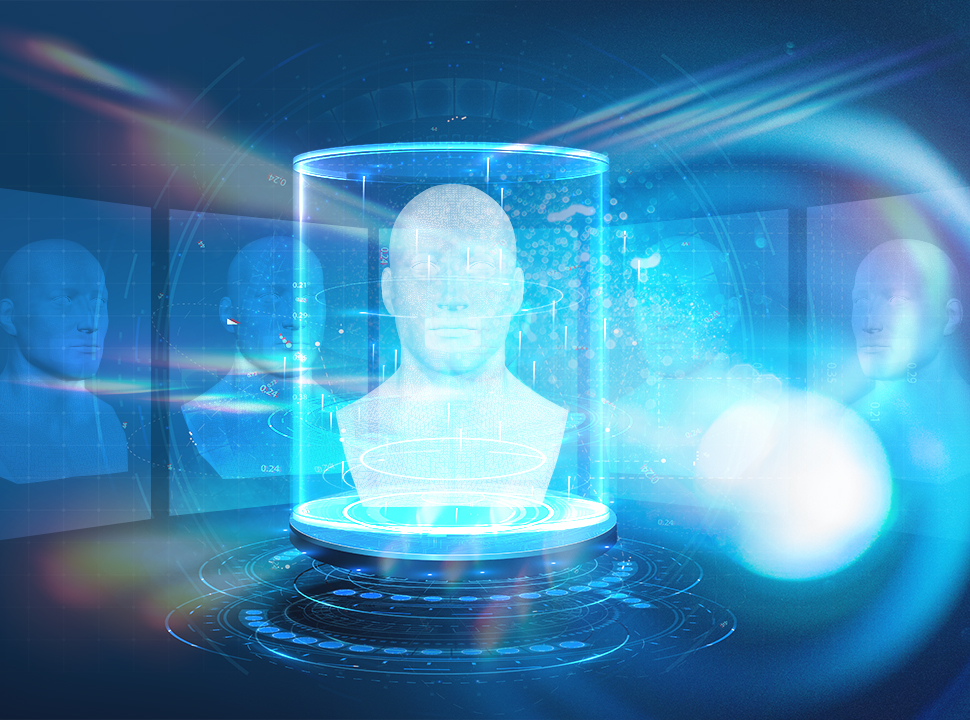
Cal-Berkeley researchers developed a single-step 3D printing process called Computed Axial Lithography.
In 2016, researchers at the University of California-Berkeley invented a 3D-printing process they named “computed axial lithography” (CAL), where solid objects can be created in essentially one step using tomographic reconstruction.
“At the time,” said Hayden Taylor, principal investigator and professor of mechanical engineering, “we were looking at various ways to increase the speed of additive manufacturing, including holographic methods, when the idea struck us to adapt the principles of computed tomography for manufacturing in various materials, including photopolymers.”
Glass, too, was of special interest to Taylor. Glass is often preferred for lenses and optical components in cameras and other imaging equipment, as well as in microfluidic devices and other diagnostic instruments. However, current manufacturing methods for these glass components are often slow and expensive and not suitable for higher-volume production.
Taylor also learned that research engineers at Albert Ludwig University in Freiburg, Germany, led by Frederik Kotz-Helmer, had developed 3D-printable glass nanocomposite materials. The special resin material containing nanoparticles of glass is surrounded by a light-sensitive binder liquid. Digital light projections from the printer solidify the binder; the printed object is then heated to remove the binder and fuse the particles together into a solid object of pure glass.
“Kotz-Helmer and his team have achieved really exciting results with their 3D-printable glass nanocomposite materials in layer-by-layer digital light printing,” said Taylor. “It was apparent that the high transparency of the glass-based materials would make them attractive for tomographic printing as well. We started collaborating with them to demonstrate the use of computed axial lithography to process these materials.”
The results were impressive. Using a modified-CAL system and these advanced materials, Taylor was able to print complete glass microstructures in a single step, with features down to about 50 millionths of a meter.
More for You: 3D Printing Goes Mobile
Taylor adjusted the design of the computed axial lithography (CAL) system so it can quickly 3D-print glass microstructures with higher optical quality, design flexibility, and strength than other additive manufacturing (AM) technologies. What’s more, unlike other AM methods that build up objects one layer at a time, CAL 3D-prints the entire object simultaneously, saving considerable time.
To achieve the creation of micro-scale features using glass nanocomposite materials, “The optical design of the printing system had to be developed significantly, including through the introduction of a laser diode light source,” said Taylor. “There was painstaking characterization of the ‘modulation transfer function’ of the optical system to ensure that features in the tens of microns could be printed. The material formulation also required close attention.”
The specific formulation of the printed silica nanocomposite precursor (to combine high transparency, low scattering, and a high contrast dose response) is crucial for tomographic printing, where light rays need to pass all the way through the printing volume.
“The key enabler for this is the binder has a refractive index that is virtually identical to that of the glass, so that light passes through the material with virtually no scattering,” said Taylor. “The CAL printing process and the glass nanocomposite materials are a perfect match for each other.”
More Additive Manufacturing: Printing Across Barries with Sound
The system uses a laser to project patterns of light into a rotating volume of light-sensitive material, building up a 3D light dose that then solidifies in the desired shape. The layerless nature of the CAL process enables smooth surfaces and complex geometries. The team carefully tuned the concentrations of the photo-initiator and a free-radical quencher molecule (TEMPO) to ensure a high-contrast response to patterned light and the ability to preserve internal voids in printed lattice structures. Post-processing of the material after exposure to light also needed refinement, as did the method for separating the printed “green” part from the surrounding, uncured, resin precursor.
Taylor also wanted to know whether the layer-free printing process of computed axial lithography would produce smoother surfaces than the typical layer-by-layer AM methods and if the greater smoothness would increase the fracture strength of printed components.
“Our results showed that, indeed, CAL printing gave significantly smoother surfaces than layer-based stereolithography [SLA] printing,” said Taylor. “We expected that because, with greater smoothness, there would be fewer and smaller flaws in the components on average and that a CAL-printed glass component be able to take a higher load before fracturing.”
What was surprising is that they did not find a significant increase in the average fracture strength of CAL-printed beams compared to SLA-printed specimens when tested under bending loads, he noted. However, the distribution of fracture loads was substantially narrower in the CAL-printed components.
Editor’s Pick: A Morphing Nozzle for the Fouth Dimension
“This is potentially valuable because it suggests that CAL may be able to produce glass components with a required probability of achieving a minimum breaking load, while using less material than other processes,” he said.
When the team first created the CAL, it could print objects into polymers with features down to about a third of a millimeter in size. Now, with micro-CAL, objects can be printed in polymers with features down to about 20 millionths of a meter, or about a quarter of a human hair’s breadth. “And for the first time, we have shown how this method can print not only into polymers but also into glass, with features down to about 50 millionths of a meter,” Taylor added.
In tandem with his work on silica glass printing, Taylor is interested in the possibilities of applying CAL to bioprinting. Because the object being printed does not move relative to the precursor material during light exposure, it opens the way to processing exceptionally low-stiffness and delicate materials, which could be damaged by the hydrodynamic stresses common in other additive processes.
The team is exploring other applications of silica glass printing, such as 3D-printed microfluidic devices for stringent operating conditions that may not be compatible with polymers. Other possibilities are micro-scale optical components for a variety of applications.
“We have also received considerable industrial interest and are optimistic for the future of this process,” Taylor said.
Mark Crawford is a science and technology writer in Corrales, N.M.
“At the time,” said Hayden Taylor, principal investigator and professor of mechanical engineering, “we were looking at various ways to increase the speed of additive manufacturing, including holographic methods, when the idea struck us to adapt the principles of computed tomography for manufacturing in various materials, including photopolymers.”
Glass, too, was of special interest to Taylor. Glass is often preferred for lenses and optical components in cameras and other imaging equipment, as well as in microfluidic devices and other diagnostic instruments. However, current manufacturing methods for these glass components are often slow and expensive and not suitable for higher-volume production.
Taylor also learned that research engineers at Albert Ludwig University in Freiburg, Germany, led by Frederik Kotz-Helmer, had developed 3D-printable glass nanocomposite materials. The special resin material containing nanoparticles of glass is surrounded by a light-sensitive binder liquid. Digital light projections from the printer solidify the binder; the printed object is then heated to remove the binder and fuse the particles together into a solid object of pure glass.
“Kotz-Helmer and his team have achieved really exciting results with their 3D-printable glass nanocomposite materials in layer-by-layer digital light printing,” said Taylor. “It was apparent that the high transparency of the glass-based materials would make them attractive for tomographic printing as well. We started collaborating with them to demonstrate the use of computed axial lithography to process these materials.”
The results were impressive. Using a modified-CAL system and these advanced materials, Taylor was able to print complete glass microstructures in a single step, with features down to about 50 millionths of a meter.
More for You: 3D Printing Goes Mobile
Taylor adjusted the design of the computed axial lithography (CAL) system so it can quickly 3D-print glass microstructures with higher optical quality, design flexibility, and strength than other additive manufacturing (AM) technologies. What’s more, unlike other AM methods that build up objects one layer at a time, CAL 3D-prints the entire object simultaneously, saving considerable time.
To achieve the creation of micro-scale features using glass nanocomposite materials, “The optical design of the printing system had to be developed significantly, including through the introduction of a laser diode light source,” said Taylor. “There was painstaking characterization of the ‘modulation transfer function’ of the optical system to ensure that features in the tens of microns could be printed. The material formulation also required close attention.”
The specific formulation of the printed silica nanocomposite precursor (to combine high transparency, low scattering, and a high contrast dose response) is crucial for tomographic printing, where light rays need to pass all the way through the printing volume.
“The key enabler for this is the binder has a refractive index that is virtually identical to that of the glass, so that light passes through the material with virtually no scattering,” said Taylor. “The CAL printing process and the glass nanocomposite materials are a perfect match for each other.”
More Additive Manufacturing: Printing Across Barries with Sound
The system uses a laser to project patterns of light into a rotating volume of light-sensitive material, building up a 3D light dose that then solidifies in the desired shape. The layerless nature of the CAL process enables smooth surfaces and complex geometries. The team carefully tuned the concentrations of the photo-initiator and a free-radical quencher molecule (TEMPO) to ensure a high-contrast response to patterned light and the ability to preserve internal voids in printed lattice structures. Post-processing of the material after exposure to light also needed refinement, as did the method for separating the printed “green” part from the surrounding, uncured, resin precursor.
Taylor also wanted to know whether the layer-free printing process of computed axial lithography would produce smoother surfaces than the typical layer-by-layer AM methods and if the greater smoothness would increase the fracture strength of printed components.
“Our results showed that, indeed, CAL printing gave significantly smoother surfaces than layer-based stereolithography [SLA] printing,” said Taylor. “We expected that because, with greater smoothness, there would be fewer and smaller flaws in the components on average and that a CAL-printed glass component be able to take a higher load before fracturing.”
What was surprising is that they did not find a significant increase in the average fracture strength of CAL-printed beams compared to SLA-printed specimens when tested under bending loads, he noted. However, the distribution of fracture loads was substantially narrower in the CAL-printed components.
Editor’s Pick: A Morphing Nozzle for the Fouth Dimension
“This is potentially valuable because it suggests that CAL may be able to produce glass components with a required probability of achieving a minimum breaking load, while using less material than other processes,” he said.
When the team first created the CAL, it could print objects into polymers with features down to about a third of a millimeter in size. Now, with micro-CAL, objects can be printed in polymers with features down to about 20 millionths of a meter, or about a quarter of a human hair’s breadth. “And for the first time, we have shown how this method can print not only into polymers but also into glass, with features down to about 50 millionths of a meter,” Taylor added.
In tandem with his work on silica glass printing, Taylor is interested in the possibilities of applying CAL to bioprinting. Because the object being printed does not move relative to the precursor material during light exposure, it opens the way to processing exceptionally low-stiffness and delicate materials, which could be damaged by the hydrodynamic stresses common in other additive processes.
The team is exploring other applications of silica glass printing, such as 3D-printed microfluidic devices for stringent operating conditions that may not be compatible with polymers. Other possibilities are micro-scale optical components for a variety of applications.
“We have also received considerable industrial interest and are optimistic for the future of this process,” Taylor said.
Mark Crawford is a science and technology writer in Corrales, N.M.