The Solar Explosion
The Solar Explosion
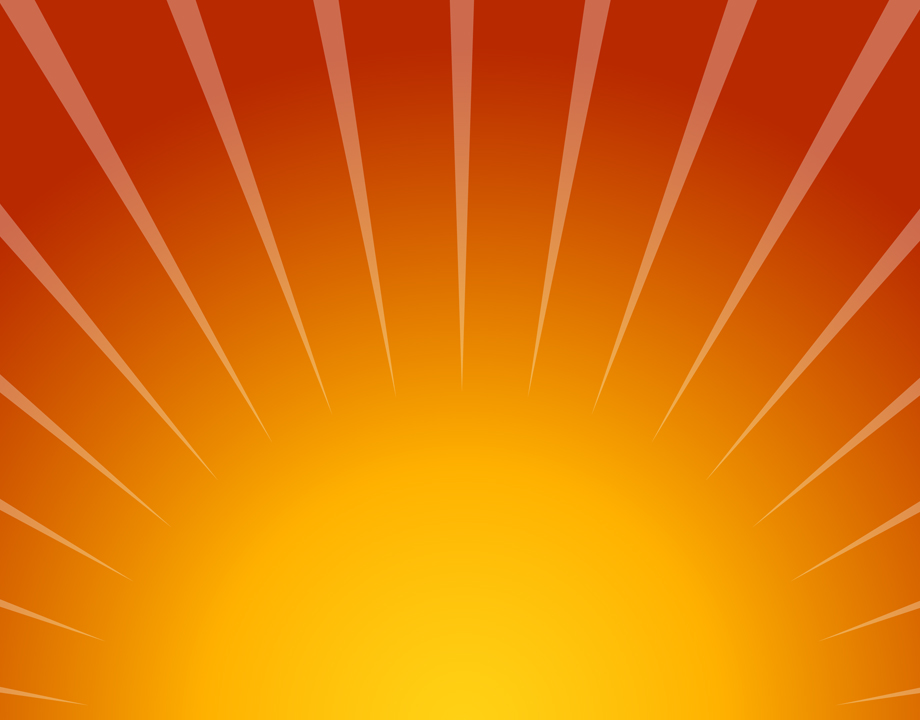
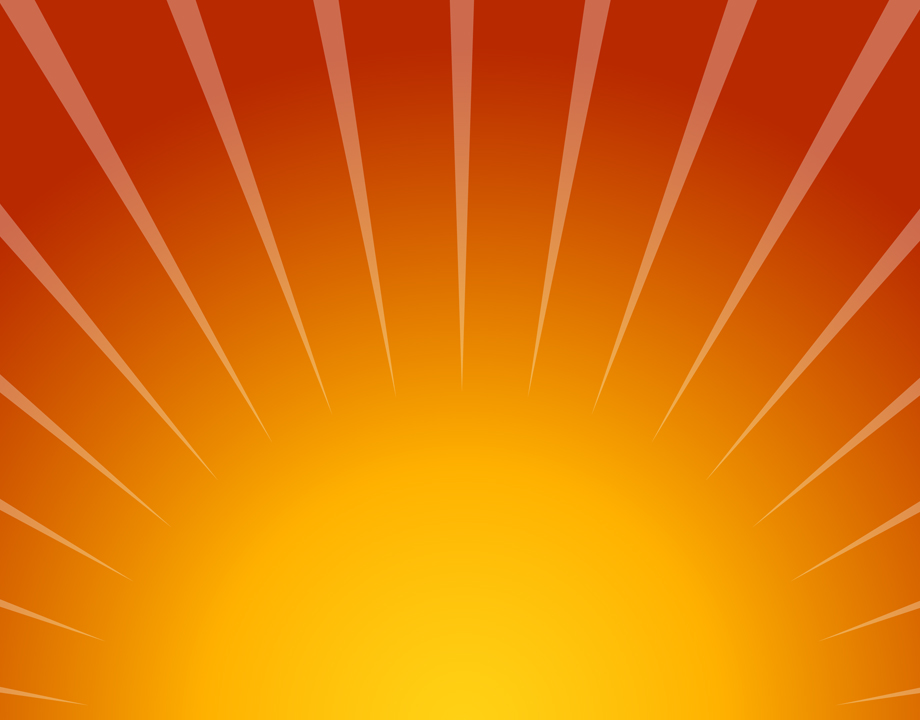
How did photovoltaics transform from being an expensive, niche electricity source to the least costly power on the grid? It took a triumph of industrial engineering.
When photovoltaic (PV) cells first burst into the public consciousness in the 1960s and 1970s, they were useful for producing electricity only in applications where no alternative could be found. Satellites in geosynchronous orbit, for instance, were too far away from Earth to refuel, and it was inconvenient to keep replacing batteries on low-power, pocket-size electronic calculators. For other applications, however, the cost of electricity from PV cells was too exorbitant to be acceptable.
In the second decade of the 21st century, the situation has been inverted. During the last five decades, the cost of PV modules dropped from $100 per peak watt (Wp) down to as low as $2/Wp in 2019 (for utility-level PV systems). In one real-world example of this trend from the past year, the Kaneland School District of Maple Park, Ill., signed a power purchase agreement to have 1,051 kW solar PV installed at three schools; the district will have no upfront costs and will buy electricity produced by the panels at $0.048 per kWh as compared to the market rate of $0.0693 per kWh.
Not only is PV power becoming cheap, but it is also now widely available. On a global basis, very few industries have expanded as quickly as PV. According to the International Energy Agency, 165 GW of PV systems came online in 2016, about two-thirds of the world’s net power generating sources.
What breakthroughs led to this veritable revolution in photovoltaics? The commonly told story is that China started manufacturing lower-quality panels and dumped them on the world market at prices near (or even below) the cost of production. The truth is more complicated. Chinese manufacturing at scale played a part, but so did German industrial policy and a focus on improving the complete power system, not just the PV cells.
In short, the revolution in photovoltaics is a triumph of engineering.
Solar power has had a futuristic sensibility for 70 years, but rudimentary photovoltaic technology dates back nearly two centuries. Edmond Becquerel first observed the phenomenon of conversion of sunlight into electricity (photovoltaic effect) back in 1839 while experimenting with two silver-coated platinum electrodes by placing them in an acidic solution and exposing one of the electrodes to sunlight. Other scientists, working in the 1870s, were able to turn light into electricity using the metal selenium.
The United States became a center of research in the mid-20th century. Gerald Pearson, Calvin Fuller, and Daryl Chapin, while working to develop silicon transistors at Bell Labs in 1954, found that silicon solar cells were much more efficient than the selenium cells, producing electricity with an efficiency of 6 percent. The first commercial application of PV cells was powering of a radio for a satellite named Vanguard that was launched in 1958 by the U.S. Naval Research Laboratory. The PV-powered communication satellite, the Bell Labs-built Telstar, was launched in 1962.
Research spurred by the oil embargoes of the 1970s was often funded by independent oil-producing companies such as Exxon, Shell, and British Petroleum. Exxon funded research by Elliot Berman of Solar Power Corp. that led to the discovery that multiple silicon crystal in place of single crystal could lower the cost of PV cells significantly.
In the early 1980s, the Federal Republic of Germany engaged in a proactive policy, including solar power price support, which catapulted PV technology development and manufacturing, albeit at a comparatively higher cost per watt produced than would normally be economic. A series of renewable energy laws—beginning with the Electricity Feed Act (Stromeinspeisungsgesetz) in 1991—required utilities to facilitate power purchase agreements (PPA) for PV systems so that the excess energy produced would be purchased. The PPA, in the form of feed-in tariffs, made it possible for the PV systems to compete with the conventional sources.
Related Story: Getting More Energy from Solar Panels
Such developments started to improve the economics of photovoltaics. According to some estimates, the increase in cell efficiency alone helped to reduce the inflation-adjusted costs by 24 percent during the period from 1980 to 2001 and 12 percent from 2001 to 2012. Other factors, such as research (around 60 percent) and mass manufacturing (around 20 percent), played critical roles in bringing down the costs.
The biggest effect of these cost reductions has been in removing the barriers to utility-scale deployment of solar power. While the output of small-scale systems has roughly tripled in recent years, data from the U.S. Energy Information Administration shows that electricity production from large grid-connected PV power plants increased from 76 million kWh in 2008 to a staggering 63 billion kWh in 2018—nearly a factor of 1,000.
The commercial-scale manufacturing of PV modules started in the United States. So why didn’t the U.S., where the solar PV technology was invented and put to practical use, continue to become the market leader? Many accounts of the breakthrough of solar power to the utility market begin—and end—with Chinese government support of manufacturers to mass produce photovoltaic modules. And it’s important to understand that part of the story.
Chinese companies started manufacturing PV equipment in the late 1990s and began to expand rapidly in the early 2000s. This was partly enabled by the German government, which was interested in developing low-cost sourcing of the PV modules to support its aggressive solar power plans and provided both technical help and financial assistance. In order to establish its presence and gain a major PV module market share, the Chinese government proactively engaged in providing direct subsidy in a variety of ways to its manufacturing units. In addition to the direct subsidies, supports were extended to provide research grants, access to low-interest loans, inexpensive land, and tax benefits.
At the early stages, China imported most of the silicon, and the equipment to manufacture PV module from other countries. The lack of knowledge in producing high-purity polycrystalline silicon was an impediment that China had to overcome in mass producing multi crystalline PV module of high quality and durability. Specifically, China was plagued with energy intensive manufacturing, scalability issues, and poorly trained workers lacking required skills. In time, however, China was able to manufacture finished modules relatively cheaply using inexpensive labor and efficient module encapsulation. Chinese manufacturers began ramping up manufacturing capacity to meet a rapacious demand for PV among China’s domestic customers, but it was so great—more than the existing worldwide demand—that it flooded the market to the extent that PV module prices fell by 75 percent.
By some analysis, this dramatic cost reduction was due not just to inexpensive labor, but also ease of capital including free loans, infrastructure, and availability of land. According to a George Washington University report, following the German model, China started offering heavily subsidized tariffs for rooftop solar systems that invigorated its domestic solar market.
Undoubtedly, China’s state-controlled economy and targeted subsidies have been major factors in overcoming the barriers, and relatively quickly China caught up with its inadequacy in both the technical knowledge of PV manufacturing and the precision machinery manufacturing of PV cells and modules. R&D laboratories were built to advance PV research in increased efficiency of cell development and module manufacturing techniques. A similar situation existed for robust silicon ingot-slicing processes and manufacturing of ultra-thin wafers.
Today, PV module production is dominated by Chinese manufacturers. The International Energy Agency reported that Chinese PV companies in 2017 produced about 60 percent of world’s PV modules while consuming around 50 percent of the global demand.
The burgeoning dominance of Chinese photovoltaic module manufacturers is certainly a major part of solar’s breakthrough into the utility-scale market. But it isn’t the only factor. While Chinese factories were able to mass produce PV modules resulting in a substantial drop in prices, Germany concentrated on developing reliable power electronics—namely the inverters for residential, commercial, and large-scale PV applications.
Germany is known for its long and cloudy winter days. Southern Germany, the region with the most sun with about 1,700 solar-hours per year, has a lower photovoltaic power potential than even the cloudiest parts of the continental United States. (The sunniest parts of the U.S., such as Yuma, Ariz., have a solar power potential that’s more than double.) With so much annual gloom, solar would seem to be an unlikely source of energy to power its homes, office buildings, and factories. And the cost of solar was exorbitant. Against all these odds, the German policy makers and the people chose solar photovoltaic.
During the 1980s, the reduction of costs in pre-unified Germany began to accelerate as the commercial-scale PV systems became widely used in offices and various comparable properties. In 1992, after unification, the German PV manufactures could offer modules at $7 per Wp; by 2008, the price was half that, thanks mostly to planned initiatives such as the 1,000 Solar Roofs Program of the early 1990s and
the 100,000 Solar Roofs Program of the early 2000s. In spite of this support, the 2008 global economic crisis had a detrimental effect on the German PV module manufacturing industry. Some plants were shuttered and others had to cut back production severely. On top of that, around 2012, Chinese modules became cost-competitive, further diminishing the business viability of the German PV module manufacturers. Nevertheless, the German PV market began to expand and the PV energy production increased from 0.2 TWh in 2002 to 28 TWh in 2012.
Germany’s deliberate move to solar provided opportunities to become a significant player in the field of photovoltaic power technologies and manufacturing beyond the simple production of PV cells. Thanks to robust research and development efforts and a trained workforce, German companies became the market leader in the area of solar PV power electronics, especially the inverters. The importance of this critical component for a PV system, vis-à-vis its stable and reliable controllability feature, is key and cannot be overemphasized.
Solar energy is an intermittent source, and stable and reliable output from a PV system—either to a dedicated load or to a grid—is critical from the utility’s availability and reliability points of view. Inverters allow electricity produced by the PV modules to be fed into the load and excess power to the grid or to the storage system.
This key component converts direct current, such as the electricity produced by photovoltaic systems, to alternating current. In photovoltaic system applications, these devices will either feed that ac onto the electric grid or store excess electricity in batteries. Inverters also feature integrated communication for individual module optimization, so that if a fault is detected by the inverter’s processor, a message will be sent to the system operator so action can be taken to rectify the problem.
German inverter manufactures introduced silicon carbide-based components in place of silicon, eliminating the need to derate power below an outdoor temperature of 50 °C, achieving enhanced power conversion efficiency and minimizing losses. And the inverters provide energy management solutions offering marketing of power both actively and reactively, as well as “shade management” functionality, thereby ensuring maximum yield in case of excessive shading.
To connect high-voltage storage systems to the inverter, Germany has developed high switching frequency, three-phase nongalvanically isolated dc-to-dc converter utilizing metal-oxide-semiconductor field-effect transistors made of silicon carbide. In addition to offering dc surge protection and upgradable ac protection, these inverters are also capable of providing communication interface protection. Monitoring portals have also been included, so an operator can observe the performance of a PV system or each of the modules without interruption, thereby offering maximum system availability facilitating ease of system planning and dispatching options.
More on Solar: New Solar Energy Device Beams Heat into Space
In 2014, approximately 1.5 million PV systems were installed throughout Germany ranging from small rooftop systems to large-scale PV power plants, including a 100-MW central PV power plant. By 2016, the total installed capacity was 41.3 GW, second only to China. Other countries—South Korea in particular—are entering the PV inverter field. In a January 2019 article, PV Magazine reported that the leading German inverter manufacture KACO sold its central inverter manufacturing technology to its Korean subsidiary while concentrating on string (PV modules wired in series) inverters and its energy storage business.
The power electronics developed and manufactured primarily in Germany has been an unsung factor the emergence of solar power. To be sure, the low-cost PV modules produced with significant government support by Chinese manufacturers have brought down the cost by dizzying amounts. But without the smart power electronics, utilizing advanced materials, a skilled workforce, and engineering prowess, it would be all but impossible for solar power to integrate itself into the electric power grid, where reliability is the watchword. Germany’s contribution allowed solar PV to take a first step toward being a mainstream source of electricity.
Clearly, the PV industry has great growth potential. If the last five decades of increased PV cell efficiency trajectory, reduced manufacturing costs, and advances in power conversion and inverter technology development is any guide, the growth should continue provided challenges such as regulatory policies to reflect changing market conditions, training of skilled professionals, and reliable grid interconnections are mitigated.
Amiyo K. Basu is an engineering consultant based in Austin, Texas. He became an ASME Fellow in 2002.
In the second decade of the 21st century, the situation has been inverted. During the last five decades, the cost of PV modules dropped from $100 per peak watt (Wp) down to as low as $2/Wp in 2019 (for utility-level PV systems). In one real-world example of this trend from the past year, the Kaneland School District of Maple Park, Ill., signed a power purchase agreement to have 1,051 kW solar PV installed at three schools; the district will have no upfront costs and will buy electricity produced by the panels at $0.048 per kWh as compared to the market rate of $0.0693 per kWh.
Not only is PV power becoming cheap, but it is also now widely available. On a global basis, very few industries have expanded as quickly as PV. According to the International Energy Agency, 165 GW of PV systems came online in 2016, about two-thirds of the world’s net power generating sources.
What breakthroughs led to this veritable revolution in photovoltaics? The commonly told story is that China started manufacturing lower-quality panels and dumped them on the world market at prices near (or even below) the cost of production. The truth is more complicated. Chinese manufacturing at scale played a part, but so did German industrial policy and a focus on improving the complete power system, not just the PV cells.
In short, the revolution in photovoltaics is a triumph of engineering.
Sunlight into Electricity
Solar power has had a futuristic sensibility for 70 years, but rudimentary photovoltaic technology dates back nearly two centuries. Edmond Becquerel first observed the phenomenon of conversion of sunlight into electricity (photovoltaic effect) back in 1839 while experimenting with two silver-coated platinum electrodes by placing them in an acidic solution and exposing one of the electrodes to sunlight. Other scientists, working in the 1870s, were able to turn light into electricity using the metal selenium.
The United States became a center of research in the mid-20th century. Gerald Pearson, Calvin Fuller, and Daryl Chapin, while working to develop silicon transistors at Bell Labs in 1954, found that silicon solar cells were much more efficient than the selenium cells, producing electricity with an efficiency of 6 percent. The first commercial application of PV cells was powering of a radio for a satellite named Vanguard that was launched in 1958 by the U.S. Naval Research Laboratory. The PV-powered communication satellite, the Bell Labs-built Telstar, was launched in 1962.
Research spurred by the oil embargoes of the 1970s was often funded by independent oil-producing companies such as Exxon, Shell, and British Petroleum. Exxon funded research by Elliot Berman of Solar Power Corp. that led to the discovery that multiple silicon crystal in place of single crystal could lower the cost of PV cells significantly.
In the early 1980s, the Federal Republic of Germany engaged in a proactive policy, including solar power price support, which catapulted PV technology development and manufacturing, albeit at a comparatively higher cost per watt produced than would normally be economic. A series of renewable energy laws—beginning with the Electricity Feed Act (Stromeinspeisungsgesetz) in 1991—required utilities to facilitate power purchase agreements (PPA) for PV systems so that the excess energy produced would be purchased. The PPA, in the form of feed-in tariffs, made it possible for the PV systems to compete with the conventional sources.
Related Story: Getting More Energy from Solar Panels
Such developments started to improve the economics of photovoltaics. According to some estimates, the increase in cell efficiency alone helped to reduce the inflation-adjusted costs by 24 percent during the period from 1980 to 2001 and 12 percent from 2001 to 2012. Other factors, such as research (around 60 percent) and mass manufacturing (around 20 percent), played critical roles in bringing down the costs.
The biggest effect of these cost reductions has been in removing the barriers to utility-scale deployment of solar power. While the output of small-scale systems has roughly tripled in recent years, data from the U.S. Energy Information Administration shows that electricity production from large grid-connected PV power plants increased from 76 million kWh in 2008 to a staggering 63 billion kWh in 2018—nearly a factor of 1,000.
The China Factor
The commercial-scale manufacturing of PV modules started in the United States. So why didn’t the U.S., where the solar PV technology was invented and put to practical use, continue to become the market leader? Many accounts of the breakthrough of solar power to the utility market begin—and end—with Chinese government support of manufacturers to mass produce photovoltaic modules. And it’s important to understand that part of the story.
Chinese companies started manufacturing PV equipment in the late 1990s and began to expand rapidly in the early 2000s. This was partly enabled by the German government, which was interested in developing low-cost sourcing of the PV modules to support its aggressive solar power plans and provided both technical help and financial assistance. In order to establish its presence and gain a major PV module market share, the Chinese government proactively engaged in providing direct subsidy in a variety of ways to its manufacturing units. In addition to the direct subsidies, supports were extended to provide research grants, access to low-interest loans, inexpensive land, and tax benefits.
At the early stages, China imported most of the silicon, and the equipment to manufacture PV module from other countries. The lack of knowledge in producing high-purity polycrystalline silicon was an impediment that China had to overcome in mass producing multi crystalline PV module of high quality and durability. Specifically, China was plagued with energy intensive manufacturing, scalability issues, and poorly trained workers lacking required skills. In time, however, China was able to manufacture finished modules relatively cheaply using inexpensive labor and efficient module encapsulation. Chinese manufacturers began ramping up manufacturing capacity to meet a rapacious demand for PV among China’s domestic customers, but it was so great—more than the existing worldwide demand—that it flooded the market to the extent that PV module prices fell by 75 percent.
By some analysis, this dramatic cost reduction was due not just to inexpensive labor, but also ease of capital including free loans, infrastructure, and availability of land. According to a George Washington University report, following the German model, China started offering heavily subsidized tariffs for rooftop solar systems that invigorated its domestic solar market.
Undoubtedly, China’s state-controlled economy and targeted subsidies have been major factors in overcoming the barriers, and relatively quickly China caught up with its inadequacy in both the technical knowledge of PV manufacturing and the precision machinery manufacturing of PV cells and modules. R&D laboratories were built to advance PV research in increased efficiency of cell development and module manufacturing techniques. A similar situation existed for robust silicon ingot-slicing processes and manufacturing of ultra-thin wafers.
Today, PV module production is dominated by Chinese manufacturers. The International Energy Agency reported that Chinese PV companies in 2017 produced about 60 percent of world’s PV modules while consuming around 50 percent of the global demand.
Industrial Policy
The burgeoning dominance of Chinese photovoltaic module manufacturers is certainly a major part of solar’s breakthrough into the utility-scale market. But it isn’t the only factor. While Chinese factories were able to mass produce PV modules resulting in a substantial drop in prices, Germany concentrated on developing reliable power electronics—namely the inverters for residential, commercial, and large-scale PV applications.
Germany is known for its long and cloudy winter days. Southern Germany, the region with the most sun with about 1,700 solar-hours per year, has a lower photovoltaic power potential than even the cloudiest parts of the continental United States. (The sunniest parts of the U.S., such as Yuma, Ariz., have a solar power potential that’s more than double.) With so much annual gloom, solar would seem to be an unlikely source of energy to power its homes, office buildings, and factories. And the cost of solar was exorbitant. Against all these odds, the German policy makers and the people chose solar photovoltaic.
During the 1980s, the reduction of costs in pre-unified Germany began to accelerate as the commercial-scale PV systems became widely used in offices and various comparable properties. In 1992, after unification, the German PV manufactures could offer modules at $7 per Wp; by 2008, the price was half that, thanks mostly to planned initiatives such as the 1,000 Solar Roofs Program of the early 1990s and
the 100,000 Solar Roofs Program of the early 2000s. In spite of this support, the 2008 global economic crisis had a detrimental effect on the German PV module manufacturing industry. Some plants were shuttered and others had to cut back production severely. On top of that, around 2012, Chinese modules became cost-competitive, further diminishing the business viability of the German PV module manufacturers. Nevertheless, the German PV market began to expand and the PV energy production increased from 0.2 TWh in 2002 to 28 TWh in 2012.
Germany’s deliberate move to solar provided opportunities to become a significant player in the field of photovoltaic power technologies and manufacturing beyond the simple production of PV cells. Thanks to robust research and development efforts and a trained workforce, German companies became the market leader in the area of solar PV power electronics, especially the inverters. The importance of this critical component for a PV system, vis-à-vis its stable and reliable controllability feature, is key and cannot be overemphasized.
Solar energy is an intermittent source, and stable and reliable output from a PV system—either to a dedicated load or to a grid—is critical from the utility’s availability and reliability points of view. Inverters allow electricity produced by the PV modules to be fed into the load and excess power to the grid or to the storage system.
This key component converts direct current, such as the electricity produced by photovoltaic systems, to alternating current. In photovoltaic system applications, these devices will either feed that ac onto the electric grid or store excess electricity in batteries. Inverters also feature integrated communication for individual module optimization, so that if a fault is detected by the inverter’s processor, a message will be sent to the system operator so action can be taken to rectify the problem.
German inverter manufactures introduced silicon carbide-based components in place of silicon, eliminating the need to derate power below an outdoor temperature of 50 °C, achieving enhanced power conversion efficiency and minimizing losses. And the inverters provide energy management solutions offering marketing of power both actively and reactively, as well as “shade management” functionality, thereby ensuring maximum yield in case of excessive shading.
To connect high-voltage storage systems to the inverter, Germany has developed high switching frequency, three-phase nongalvanically isolated dc-to-dc converter utilizing metal-oxide-semiconductor field-effect transistors made of silicon carbide. In addition to offering dc surge protection and upgradable ac protection, these inverters are also capable of providing communication interface protection. Monitoring portals have also been included, so an operator can observe the performance of a PV system or each of the modules without interruption, thereby offering maximum system availability facilitating ease of system planning and dispatching options.
More on Solar: New Solar Energy Device Beams Heat into Space
In 2014, approximately 1.5 million PV systems were installed throughout Germany ranging from small rooftop systems to large-scale PV power plants, including a 100-MW central PV power plant. By 2016, the total installed capacity was 41.3 GW, second only to China. Other countries—South Korea in particular—are entering the PV inverter field. In a January 2019 article, PV Magazine reported that the leading German inverter manufacture KACO sold its central inverter manufacturing technology to its Korean subsidiary while concentrating on string (PV modules wired in series) inverters and its energy storage business.
The power electronics developed and manufactured primarily in Germany has been an unsung factor the emergence of solar power. To be sure, the low-cost PV modules produced with significant government support by Chinese manufacturers have brought down the cost by dizzying amounts. But without the smart power electronics, utilizing advanced materials, a skilled workforce, and engineering prowess, it would be all but impossible for solar power to integrate itself into the electric power grid, where reliability is the watchword. Germany’s contribution allowed solar PV to take a first step toward being a mainstream source of electricity.
Clearly, the PV industry has great growth potential. If the last five decades of increased PV cell efficiency trajectory, reduced manufacturing costs, and advances in power conversion and inverter technology development is any guide, the growth should continue provided challenges such as regulatory policies to reflect changing market conditions, training of skilled professionals, and reliable grid interconnections are mitigated.
Amiyo K. Basu is an engineering consultant based in Austin, Texas. He became an ASME Fellow in 2002.