Engineers Tackle Water Shortages
Engineers Tackle Water Shortages
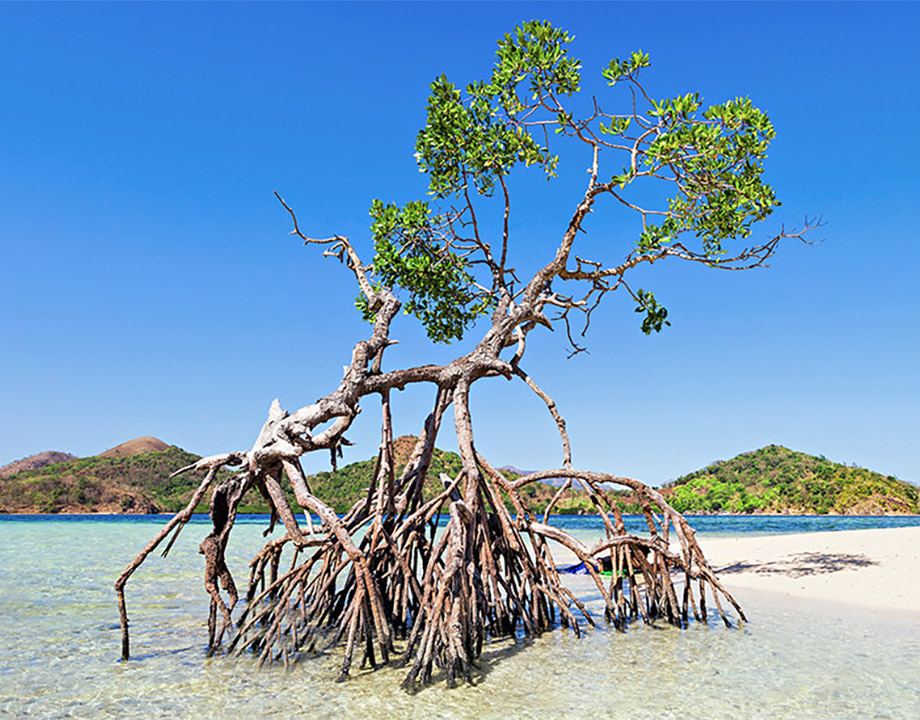
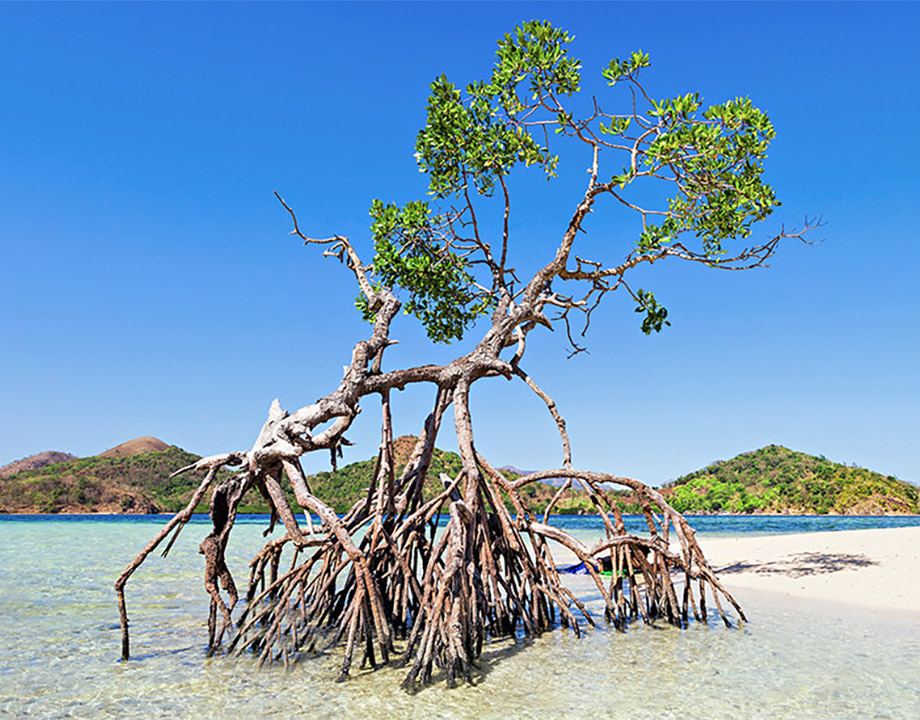
A cypress tree can desalinate brackish water and thrive. Water shortages may force humans to do the same. Photo: Yale
It’s 90 degrees by early morning. The sun is relentless, shade elusive, and the nearest source of clean water a half-hour’s walk distant. But children need bathing. There’s laundry to be washed. Food needs to be safely prepared and cooked.
That’s daily life for millions of people. Without water, no one can survive.
Globally, some two billion people struggle every day for access to a safe and ready supply of this increasingly valuable liquid. The Global Water Institute estimates 700 million people will have to move due to water scarcity over the next 10 years. Even in the United States, an estimated two million Americans face this basic challenge.
It’s an urgent problem, and scientists are developing a wide variety of technologies to solve it. In fact, many have been working for decades on finding better and more economical ways to turn non-traditional sources of water into potable water for drinking and cooking.
Now, thanks to the largest U.S. Department of Energy grant program in 60 years, announced in February 2020, those researchers will be able to compete for $100 million in funding as members of a new National Alliance for Water Innovation. NAWI will also include an additional $34 million in cost-share contributions from public and private stakeholders.
NAWI will focus on early-stage research and development of energy-efficient and cost-competitive technologies to tap nontraditional water sources for a variety of uses. Its goal is use new technology to create a “circular water economy,” one in which water is used, recycled, and reused and returned to the system to use again. Its goal is to develop technologies that enable us to reuse 90 percent of nontraditional water sources—seawater, brackish water, and industrial and agricultural wastewater—at costs similar to existing water sources, and to do it all within 10 years.
Headquartered at Lawrence Berkeley National Laboratory in Berkeley, Calif., it includes four DOE national labs, 10 industry partners, 19 U.S. research universities, a Research Advisory Council, an Industry Advisory Council, and the NAWI Alliance, an open-membership organization. Its academic partners include Arizona State University, University of California, Berkeley, Carnegie Mellon University, Georgia Tech, New Mexico State, Stanford, and University of Cincinnati—and some of the field’s most widely admired water researchers, like Menachem Elimelech at Yale.
The five-year research program is designed to invite competing proposals. The plan gives Elimelech pause, because he worries about not taking risks.
“The risk is that people will do what is comfortable,” he said. “It will be a waste of money if we don’t do something as radical as reverse osmosis. We have to think unselfishly.”
A long-time prolific researcher who published 28 papers in 2019 alone, Elimelech has strong feelings on what progress needs to be made, and the structural challenges that tend to favor scientific research over engineering.
Editor's Pick: This Robot’s Mission is to Solve the World’s Water Crisis
“Academia and the journals change the world for the worse,” he said. “The journals focus on fancy materials and ‘sparkly’ publications. In academia, they want us to publish these in the big-name publications and they judge us on our ‘sparkly’ papers. Well, most of the progress in this field is in process design, and that’s not cool. If you focus on that, you won’t get tenure. It’s a problem to overcome that’s very, very challenging.”
“What I am excited about most right now is selectivity in membranes (to desalinate water),” he said. “It’s very, very difficult and very interesting. We are trying to understand and develop processes to separate lithium from sodium, trying to develop a membrane that can distinguish between ions. We started about a year ago and are learning a lot, collaborating with many teams, like those that do molecular simulations.”
Cleaning any kind of tainted water means removing a wide array of chemicals now discarded as waste. Some, like lithium, offer significant potential commercial value if recaptured from process water.
“Lithium nowadays is very, very expensive to remove,” Elimelech said. “So it would be great to capture it and then re-sell it, but these systems are not in use now.”
Bruce Rittmann, director of the Swette Center for Environmental Biotechnology at Arizona State University’s Biodesign Institute, agrees. In fact, he is working with tainted water in his lab as well.
“What we’re working on now is mining wastes and toxic wastes, looking at valuable extractions that get precipitated into solids like silver, gold, palladium, and platinum, which are super high value,” he said. “We can get a lot of value from the things we recover, but we have to find markets for them and then we’re competing against established [vendors].”
Rittmann’s work focuses on using biofilms—colonies of mixed bacteria that grow on surfaces and often cause fouling—to remediate contaminated waters. Understanding the interactions between these different types of microbes is not simple.
“They are very small and all look alike because they’re hard to see,” he said. “But we can now integrate their genetic information and that was a giant step. Before, we could only see their ribosomes, but today we can assess their RNA, their DNA, all their genes and proteins. We can work with a full color palette.”
This gives him a better handle on what, exactly, is living in the colonies he places in a membrane biofilm reactor, which he invented. He feeds the bacteria with hydrogen gas. As the biofilms grow, they reduce oxidized contaminants and turn them into harmless substances. This sounds suspiciously like something that would work only on a lab bench, but Rittmann has scaled and commercialized the system.
“It’s used right now to treat contaminated groundwaters,” he said. “The organisms reduce nitrates like those found in fertilizers into nitrogen gas.”
The system also works with sewage, perchlorates, selenates (from coal), chromate, and chlorinated solvents. Municipal water systems in southern California have been using it for five years, Rittmann said.
Recommended: A Cheap Way to Convert Vapor into Fresh Drinking Water
NAWI has chosen environmental engineer Pedro Alvarez to lead research into resilient water transport and storage, one of six initial problems the alliance will tackle. Alvarez, a professor of civil and environmental engineering, directs Rice University’s Nanotechnology-Enabled Water Treatment Center. While most water plants are enormous, NEWT has made significant strides in the development of smaller, decentralized, fit-for-purpose systems for drinking water and industrial wastewater treatment, including off-grid solar desalination technology.
Alvarez’s research also focuses on chemicals that seep into groundwater from agricultural, industrial, and consumer products. He is especially worried about perfluorinated chemicals used for stain, water, and grease-resistant coatings, which he calls “the new PCBs.”
Yet perfluorinated compounds are only part of the problem, he said. New pollutants show up every year, creating complex brews of chemicals in aquifers that municipalities and industry must remove before they can use the water.
“Sometimes, it’s overwhelming to keep track when 10,000 new chemicals a year are introduced,” Alvarez said.
Still, his goal is to meet water purity regulations at the lowest possible cost. One recent line of research involves biofilms. While they may be benign inside a bioreactor, they can harbor disease-causing bacteria in water mains. Water works often try to remove them with disinfectants, but the biofilms protect themselves with a slimy surface layer that is hard to penetrate. The disinfectants, meanwhile, breakdown into harmful by-products.
Alvarez’s approach combines nanotechnology with biology. He starts with phages, viruses that attack and kill bacteria, and combines them with nanoclusters of carbon, sulfur, and iron oxide. Using a magnetic field, he pushes the clusters well into the biofilm, leaving the phages free to infect and kill any bacteria. In early tests, this approach killed 90 percent of bacteria without releasing any harmful disinfectant by-products.
At Rice, the emphasis is on water availability. There, Naomi Halas, who heads the Smalley-Curl Institute and her own Laboratory for Nanophotonics, is using carbon black—a cheap material used in tires to conduct heat away from the tread—to desalinate water.
Desalination is an increasingly critical technology in coastal areas with growing populations, desert climates, or polluted water. There are more than 16,000 desalination plants worldwide. Older ones use thermal technology to boil water while newer ones use membranes to separate pure water from brine. Both are expensive to build and energy-hungry to run.
Halas is among the researchers looking to build smaller desalination and purification units portable enough to load on the back of a truck and travel where they are needed most.
She starts with carbon black. Carbon black nanoparticles excel at turning sunlight into heat. Halas often demonstrates this by putting some in a glass of ice water, where they immediately start generating steam. By running sea water between a carbon black heat-transmitting surface and a membrane, she produces steam that crosses the membrane and is removed as fresh water.
“What’s exciting about this work and this research is we can extract energy from sunlight and get a more efficient footprint and output,” she said.
But Halas, like other researchers, is frustrated by the lack of funding to expand and commercialize their work. While angel investors and venture capitalists line up to fund Silicon Valley projects, those working on water treatment find financing to scale up prototypes extremely elusive. Most VCs, she said, want to invest in a software company that they can sell for a profit after two years. Scaling up water technology is a long-term commitment.
“It’s a problem with applied engineering,” she added. “You have to understand the basic physics to get to the engineering level and build a lab prototype. But the water industry wants a high throughput [commercial design] or they won’t talk to us.”
No matter how promising her work, Halas says it’s still very much in the ‘Model T’ stage.
“Water treatment and water treatment systems people don’t think of possibilities like off-grid treatment solutions or using sunlight at all,” she said. “New technology requires new companies. The water companies want things that are really incremental, but the National Science Foundation doesn’t want incremental—it wants transformative!”
Peter Fiske, executive director of NAWI, understands why water executives hesitate. “Water is regulated as a public utility, so people are very, very cautious,” he said. “Modularity is the new frontier. What if we had a new tool kit of modular compact systems that didn’t require a licensed operator? That’s where we going to see innovation.”
Learn More: Technology Brings Clean Water and Nutrition to Central America
At MIT, Evelyn Wang, who chairs the Department of Mechanical Engineering, is also working on harvesting clean water from nontraditional sources—the driest of desert air.
The system is based on a family of high-surface-area materials called metal-organic frameworks developed by University of California chemist Omar Yaghi. Wang teamed with Yaghi to create a device that can extract pure water from air with relative humidity as low as 10 percent. To put that into perspective, the Sahara Desert has an average relative humidity of 25 percent. The Gobi Desert, which is more arid, averages 12 percent.
The system is based on MOFs, which are crystals with a twist: They are nearly all air. They look something like Tinkertoys, with metal ion cluster hubs connected to one another by long organic molecules. This enables air to move through them. As it does, water molecules are attracted to the ionically charged hubs. When the hubs are saturated, the system uses heat to release the water as a vapor that it condenses into liquid.
Wang and Yaghi’s teams tested the device on a rooftop at Arizona State University in Tempe. “This is a location representative of arid areas, and it showed that we can actually harvest the water, even in subzero dewpoints,” Wang said.
Mass-spectrometer testing showed nothing from the MOF leached into the water. “It shows the material is indeed very stable, and we can get high-quality water,” Wang said.
“We believe with further development we can reach an economical price point,” Wang said. She believes she can find a market for a system that supplies 20 liters of household drinking, cooking, and washing water daily.
Yet despite new government funding and many promising technologies, serious obstacles remain to broader commercial and consumer use.
“In the marketplace, price is king,” Robert Stirling, a technoeconomic analyst who works with Rittmann and Westerhoff at Arizona State, said. “The lingua franca is dollars and cents, and we can’t bridge that gap. One of the challenges is needing to find an immediate customer.”
That customer may eventually come from people in developing countries where pure water is scarce. Joshua Briemberg, regional director for the charity WaterAid America, sees those needs firsthand while working in Chile, Colombia, and Nicaragua.
“I’ve spent at least the last five or six years looking at the applicability of technology,” said Briemberg, who graduated McGill University as a chemical engineer and has worked in water treatment and sanitation since 1991.
Briemberg knows all the ways even the best new solutions can fail. They may demand an unacceptable break with tradition. They may fail to address local environmental, legal, and institutional issues. They may require too much technological know-how to maintain and operate. And the technology itself may not work reliably.
He points to an innovative windmill-driven desalination system in Colombia as an example. The United Nations Development Program deployed 35 of these seemingly sustainable devices. After one year, only one was still working.
“The key will be the sizing of these solutions and making them accessible to individual families,” he said, from his office in Managua. “They need small, resilient, multiple solutions. There is no best solution. It’s all context based.”
It’s a big challenge. But with some cities, such as Cape Town, Mexico City, Cairo, and Tokyo, confronting water shortages and pollution rising everywhere, it is one that engineers must face—and solve.
Caitlin Kelly is a freelance writer in New York City.
That’s daily life for millions of people. Without water, no one can survive.
Globally, some two billion people struggle every day for access to a safe and ready supply of this increasingly valuable liquid. The Global Water Institute estimates 700 million people will have to move due to water scarcity over the next 10 years. Even in the United States, an estimated two million Americans face this basic challenge.
It’s an urgent problem, and scientists are developing a wide variety of technologies to solve it. In fact, many have been working for decades on finding better and more economical ways to turn non-traditional sources of water into potable water for drinking and cooking.
Now, thanks to the largest U.S. Department of Energy grant program in 60 years, announced in February 2020, those researchers will be able to compete for $100 million in funding as members of a new National Alliance for Water Innovation. NAWI will also include an additional $34 million in cost-share contributions from public and private stakeholders.
NAWI will focus on early-stage research and development of energy-efficient and cost-competitive technologies to tap nontraditional water sources for a variety of uses. Its goal is use new technology to create a “circular water economy,” one in which water is used, recycled, and reused and returned to the system to use again. Its goal is to develop technologies that enable us to reuse 90 percent of nontraditional water sources—seawater, brackish water, and industrial and agricultural wastewater—at costs similar to existing water sources, and to do it all within 10 years.
Headquartered at Lawrence Berkeley National Laboratory in Berkeley, Calif., it includes four DOE national labs, 10 industry partners, 19 U.S. research universities, a Research Advisory Council, an Industry Advisory Council, and the NAWI Alliance, an open-membership organization. Its academic partners include Arizona State University, University of California, Berkeley, Carnegie Mellon University, Georgia Tech, New Mexico State, Stanford, and University of Cincinnati—and some of the field’s most widely admired water researchers, like Menachem Elimelech at Yale.
The five-year research program is designed to invite competing proposals. The plan gives Elimelech pause, because he worries about not taking risks.
Capturing Value
“The risk is that people will do what is comfortable,” he said. “It will be a waste of money if we don’t do something as radical as reverse osmosis. We have to think unselfishly.”
A long-time prolific researcher who published 28 papers in 2019 alone, Elimelech has strong feelings on what progress needs to be made, and the structural challenges that tend to favor scientific research over engineering.
Editor's Pick: This Robot’s Mission is to Solve the World’s Water Crisis
“Academia and the journals change the world for the worse,” he said. “The journals focus on fancy materials and ‘sparkly’ publications. In academia, they want us to publish these in the big-name publications and they judge us on our ‘sparkly’ papers. Well, most of the progress in this field is in process design, and that’s not cool. If you focus on that, you won’t get tenure. It’s a problem to overcome that’s very, very challenging.”
“What I am excited about most right now is selectivity in membranes (to desalinate water),” he said. “It’s very, very difficult and very interesting. We are trying to understand and develop processes to separate lithium from sodium, trying to develop a membrane that can distinguish between ions. We started about a year ago and are learning a lot, collaborating with many teams, like those that do molecular simulations.”
Cleaning any kind of tainted water means removing a wide array of chemicals now discarded as waste. Some, like lithium, offer significant potential commercial value if recaptured from process water.
“Lithium nowadays is very, very expensive to remove,” Elimelech said. “So it would be great to capture it and then re-sell it, but these systems are not in use now.”
Bruce Rittmann, director of the Swette Center for Environmental Biotechnology at Arizona State University’s Biodesign Institute, agrees. In fact, he is working with tainted water in his lab as well.
“What we’re working on now is mining wastes and toxic wastes, looking at valuable extractions that get precipitated into solids like silver, gold, palladium, and platinum, which are super high value,” he said. “We can get a lot of value from the things we recover, but we have to find markets for them and then we’re competing against established [vendors].”
Rittmann’s work focuses on using biofilms—colonies of mixed bacteria that grow on surfaces and often cause fouling—to remediate contaminated waters. Understanding the interactions between these different types of microbes is not simple.
“They are very small and all look alike because they’re hard to see,” he said. “But we can now integrate their genetic information and that was a giant step. Before, we could only see their ribosomes, but today we can assess their RNA, their DNA, all their genes and proteins. We can work with a full color palette.”
This gives him a better handle on what, exactly, is living in the colonies he places in a membrane biofilm reactor, which he invented. He feeds the bacteria with hydrogen gas. As the biofilms grow, they reduce oxidized contaminants and turn them into harmless substances. This sounds suspiciously like something that would work only on a lab bench, but Rittmann has scaled and commercialized the system.
“It’s used right now to treat contaminated groundwaters,” he said. “The organisms reduce nitrates like those found in fertilizers into nitrogen gas.”
The system also works with sewage, perchlorates, selenates (from coal), chromate, and chlorinated solvents. Municipal water systems in southern California have been using it for five years, Rittmann said.
Recommended: A Cheap Way to Convert Vapor into Fresh Drinking Water
NAWI has chosen environmental engineer Pedro Alvarez to lead research into resilient water transport and storage, one of six initial problems the alliance will tackle. Alvarez, a professor of civil and environmental engineering, directs Rice University’s Nanotechnology-Enabled Water Treatment Center. While most water plants are enormous, NEWT has made significant strides in the development of smaller, decentralized, fit-for-purpose systems for drinking water and industrial wastewater treatment, including off-grid solar desalination technology.
Alvarez’s research also focuses on chemicals that seep into groundwater from agricultural, industrial, and consumer products. He is especially worried about perfluorinated chemicals used for stain, water, and grease-resistant coatings, which he calls “the new PCBs.”
Yet perfluorinated compounds are only part of the problem, he said. New pollutants show up every year, creating complex brews of chemicals in aquifers that municipalities and industry must remove before they can use the water.
“Sometimes, it’s overwhelming to keep track when 10,000 new chemicals a year are introduced,” Alvarez said.
Still, his goal is to meet water purity regulations at the lowest possible cost. One recent line of research involves biofilms. While they may be benign inside a bioreactor, they can harbor disease-causing bacteria in water mains. Water works often try to remove them with disinfectants, but the biofilms protect themselves with a slimy surface layer that is hard to penetrate. The disinfectants, meanwhile, breakdown into harmful by-products.
Alvarez’s approach combines nanotechnology with biology. He starts with phages, viruses that attack and kill bacteria, and combines them with nanoclusters of carbon, sulfur, and iron oxide. Using a magnetic field, he pushes the clusters well into the biofilm, leaving the phages free to infect and kill any bacteria. In early tests, this approach killed 90 percent of bacteria without releasing any harmful disinfectant by-products.
Desalination
At Rice, the emphasis is on water availability. There, Naomi Halas, who heads the Smalley-Curl Institute and her own Laboratory for Nanophotonics, is using carbon black—a cheap material used in tires to conduct heat away from the tread—to desalinate water.
Desalination is an increasingly critical technology in coastal areas with growing populations, desert climates, or polluted water. There are more than 16,000 desalination plants worldwide. Older ones use thermal technology to boil water while newer ones use membranes to separate pure water from brine. Both are expensive to build and energy-hungry to run.
Halas is among the researchers looking to build smaller desalination and purification units portable enough to load on the back of a truck and travel where they are needed most.
She starts with carbon black. Carbon black nanoparticles excel at turning sunlight into heat. Halas often demonstrates this by putting some in a glass of ice water, where they immediately start generating steam. By running sea water between a carbon black heat-transmitting surface and a membrane, she produces steam that crosses the membrane and is removed as fresh water.
“What’s exciting about this work and this research is we can extract energy from sunlight and get a more efficient footprint and output,” she said.
But Halas, like other researchers, is frustrated by the lack of funding to expand and commercialize their work. While angel investors and venture capitalists line up to fund Silicon Valley projects, those working on water treatment find financing to scale up prototypes extremely elusive. Most VCs, she said, want to invest in a software company that they can sell for a profit after two years. Scaling up water technology is a long-term commitment.
“It’s a problem with applied engineering,” she added. “You have to understand the basic physics to get to the engineering level and build a lab prototype. But the water industry wants a high throughput [commercial design] or they won’t talk to us.”
No matter how promising her work, Halas says it’s still very much in the ‘Model T’ stage.
“Water treatment and water treatment systems people don’t think of possibilities like off-grid treatment solutions or using sunlight at all,” she said. “New technology requires new companies. The water companies want things that are really incremental, but the National Science Foundation doesn’t want incremental—it wants transformative!”
Peter Fiske, executive director of NAWI, understands why water executives hesitate. “Water is regulated as a public utility, so people are very, very cautious,” he said. “Modularity is the new frontier. What if we had a new tool kit of modular compact systems that didn’t require a licensed operator? That’s where we going to see innovation.”
Learn More: Technology Brings Clean Water and Nutrition to Central America
At MIT, Evelyn Wang, who chairs the Department of Mechanical Engineering, is also working on harvesting clean water from nontraditional sources—the driest of desert air.
The system is based on a family of high-surface-area materials called metal-organic frameworks developed by University of California chemist Omar Yaghi. Wang teamed with Yaghi to create a device that can extract pure water from air with relative humidity as low as 10 percent. To put that into perspective, the Sahara Desert has an average relative humidity of 25 percent. The Gobi Desert, which is more arid, averages 12 percent.
The system is based on MOFs, which are crystals with a twist: They are nearly all air. They look something like Tinkertoys, with metal ion cluster hubs connected to one another by long organic molecules. This enables air to move through them. As it does, water molecules are attracted to the ionically charged hubs. When the hubs are saturated, the system uses heat to release the water as a vapor that it condenses into liquid.
Wang and Yaghi’s teams tested the device on a rooftop at Arizona State University in Tempe. “This is a location representative of arid areas, and it showed that we can actually harvest the water, even in subzero dewpoints,” Wang said.
Mass-spectrometer testing showed nothing from the MOF leached into the water. “It shows the material is indeed very stable, and we can get high-quality water,” Wang said.
“We believe with further development we can reach an economical price point,” Wang said. She believes she can find a market for a system that supplies 20 liters of household drinking, cooking, and washing water daily.
Yet despite new government funding and many promising technologies, serious obstacles remain to broader commercial and consumer use.
“In the marketplace, price is king,” Robert Stirling, a technoeconomic analyst who works with Rittmann and Westerhoff at Arizona State, said. “The lingua franca is dollars and cents, and we can’t bridge that gap. One of the challenges is needing to find an immediate customer.”
That customer may eventually come from people in developing countries where pure water is scarce. Joshua Briemberg, regional director for the charity WaterAid America, sees those needs firsthand while working in Chile, Colombia, and Nicaragua.
“I’ve spent at least the last five or six years looking at the applicability of technology,” said Briemberg, who graduated McGill University as a chemical engineer and has worked in water treatment and sanitation since 1991.
Briemberg knows all the ways even the best new solutions can fail. They may demand an unacceptable break with tradition. They may fail to address local environmental, legal, and institutional issues. They may require too much technological know-how to maintain and operate. And the technology itself may not work reliably.
He points to an innovative windmill-driven desalination system in Colombia as an example. The United Nations Development Program deployed 35 of these seemingly sustainable devices. After one year, only one was still working.
“The key will be the sizing of these solutions and making them accessible to individual families,” he said, from his office in Managua. “They need small, resilient, multiple solutions. There is no best solution. It’s all context based.”
It’s a big challenge. But with some cities, such as Cape Town, Mexico City, Cairo, and Tokyo, confronting water shortages and pollution rising everywhere, it is one that engineers must face—and solve.
Caitlin Kelly is a freelance writer in New York City.