Engineers Find New Uses for Gas Turbines
Engineers Find New Uses for Gas Turbines
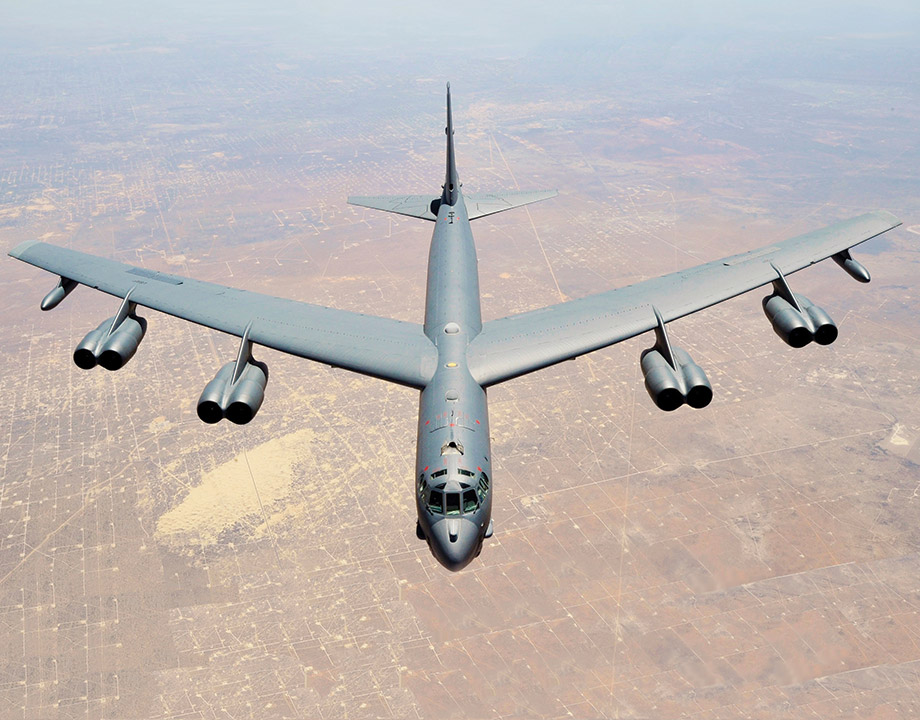
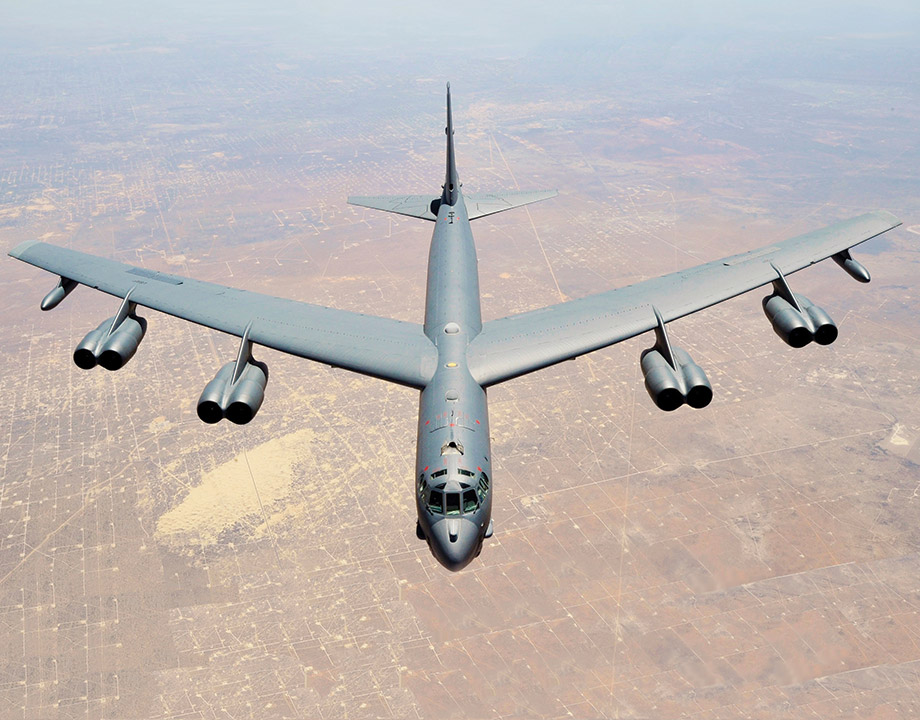
The U.S. Air Force plans to replace the jet engines in B-52H bombers with commercial versions. The move will extend the service life of these aging aircraft. Photo: U.S. Air Force
Age is relative. A five-year-old smart phone can be obsolete while a ten-year-old bottle of wine is ready to drink. This dichotomy can be seen in military aircraft, too. While militaries in several nations are taking deliveries of the F-35 Joint Strike Fighter—perhaps the most high-tech and most controversial aircraft in the world—strategists contend that the plane is already outdated and are looking ahead to the next generation of fighter aircraft. Meanwhile, the U.S. Air Force is keeping its fleet of B-52Hs in the air to the 2050s, around 90 years after the first Stratofortresses entered active service.
That plan hinges on swapping in commercial jet engines for the existing 17,000 pound-thrust TF-33 engines, which were designed in the late 1950s. As such, the airframes would be ancient by aviation standards, so much so that three generations of airmen may have served on the same bomber, but the engines would be state of the art. Does that make the planes old, or new?
Those TF-33s were designed a mere 20 years after the development of the first gas turbine in 1939. As prime movers go, gas turbines are virtually brand new, compared to, say, wind and water turbines which have been around for millennia. But they have also reached a considerable level of maturity. Gas turbines now dominate both the world’s aircraft propulsion and a good portion of electric power generation.
The fortunes of the industry are not uniform, however. The commercial jet engine market is robust and growing; the military jet engine, electric power, and other markets have been relatively flat or declining. But those are the sectors where the possibilities lie. They aren’t new, but they have the potential for renewal.
Aviation Dominates
Perhaps the best overview of the worldwide gas turbine market uses data from Forecast International, a market research firm in Newtown, Conn. Analyst Stuart Slade has tapped FI’s computer models and extensive database to calculate the value of gas turbine manufacturing production from 2009 to 2018, and has projected those values to 2033. (FI considers value of production figures to be more accurate than reported sales figures.)
Slade reports that the value of production for all gas turbines worldwide for 2018 was $86.5 billion, slightly down from $87.6 billion in 2017. As in years past, the largest portion of production values is in the aviation market -- $74.5 billion. Not only did the market grow at 3 percent from the 2017 level, but the value of production is forecast to grow to $92.2 billion by 2033.
Just as aviation dominates the total market, commercial jet engine manufacturing dominates aviation, with $66.1 billion in value of production in 2018. The production of jet engines follows the fortunes of its main customers, and the recent boom in commercial aviation has been so pronounced that over the past three years, the airline industry had generated more profit than the previous 30 years combined.
You May Also Like: Running in Place
Airlines have been clamoring for planes that use new, fuel-efficient engines, which has resulted in the popularity of the twin-engined Boeing 737 MAX and Airbus A320neo. To meet that demand, CFM International, a joint venture of GE Aviation and Safran Aircraft Engines, produced some 1,100 LEAP turbofan engine for such airliners last year, while Pratt & Whitney produced 750 of its PW 1000G series of geared turbofans. With more than 10,000 of those two airliners on order, both engine makers will be producing these engines for years to come.
The third major jet engine marker, Rolls-Royce, had some production issues in 2018 with their Trent 7000 engine, which at 70,000 lbt powers the Airbus A330neo, as well as durability problems with the Trent 1000 on Boeing’s 787. As a result, Rolls will not be bidding on an engine for Boeing’s New Midsize Airplane, the NMA, leaving the field to GE and Pratt & Whitney.
Narrow-body, single-aisle jets are the most profitable commercial aircraft, but the wide-body, twin-aisle airliner market still carries prestige. Those large planes are designed to cross oceans and need much larger engines than the 20,000-30,000 pound thrust LEAPs and PW 1000Gs. To give a sense of what the biggest planes require, General Electric will be flight testing their 100,000 lbt, GE9X Turbofan engine with an inlet fan diameter of 134 inches—just over 11 feet and nearly as wide as an A320 fuselage. The GE9X is intended to power Boeing’s new 777X, a twin-engine aircraft designed to carry more than 400 passengers.
In contrast to the boom in commercial jet engines, the other segment of the aviation market—jet engines for military aircraft—saw a slight decrease in value of production in 2018, to $8.4 billion. As noted above, some military airframes may start being re-engined with off-the-shelf commercial jets. But the specifications for military aircraft are so demanding that the technological advances needed to meet those specs filter down to both commercial jets and non-aviation gas turbines. Often, what’s revolutionary in those applications is old hat for military engines.
Most Efficient Heat Engines
The non-aviation market is not only considerably smaller than the aviation market, it’s more fragmented. Forecast International breaks out mechanical drive gas turbines, used extensively along natural gas pipelines to power compression stations, marine power gas turbines designed for ships, and microturbines are found in combined heat and power systems. The value of production for those three segments was $2.5 billion, compared to $9.5 billion for the fourth industry segment, gas turbines used in electric power generation.
The electric power segment has seen better days. In 2009, the total value of production was more than $20 billion, and it still more than $13 billion in 2017. Even looking out to 2033, Forecast International sees the value of production only partially recovering, reaching $12.3 billion.
The first gas turbine for use in electric generation was first tested 80 years ago at the Baden works of the Swiss company, Brown Boveri. Later it was installed at Neuchâtel, a city to the west of Baden. Burning fuel oil, it had a thermal efficiency of 18 percent and was used to power an electrical generator with an output up to 4 MW.
Read more about Unique Gas Turbine Engine Powering Quieter Drones
Today, simple-cycle gas turbines can reach efficiencies above 40 percent in units that generate as much as 500 MW. When matched with systems that use the high-temperature gas turbine exhaust to generate steam to run a Rankine-cycle turbine, combined-cycle gas turbines can reach thermal efficiencies as high as 60 percent—double that of most existing coal-fired plants—and power outputs greater than 1,000 MW. (As a mechanical engineer, I can’t resist repeatedly pointing out that gas turbines make up part of the most efficient heat engines yet perfected by mankind!)
These gas turbine combined-cycle (GTCC) plants also have low capital costs, in the range of $700 to $1,000 per kW, compared to $3,000 per kW for coal or $6,000 per kW for nuclear plants.
With record efficiency and low costs, GTCC plants were expected to be in high demand. But in 2018 both GE and Siemens, major gas turbine OEMs, cut their workforce significantly. It turned out that as larger and more efficient gas turbine plants have been installed, the need for more gas turbines was reduced. For various market reasons some gas turbine plants that were purchased in previous years have been mothballed, leading to a bow-wave of reserve capacity, limiting immediate demand.
Another factor leading to the recent downturn are market uncertainties caused by the introduction of increasingly cheap power from wind turbines and photovoltaic solar cells. Although these so-called renewable sources have depressed demand for new gas turbine power plants, over the long term, the intermittent nature of those facilities could increase the need for quick start gas turbine plants to back up the renewables when the sun doesn’t shine and the wind falters.
Crash Course
In an effort to decarbonize the production of electrical power, nations and large regions have added large amounts of wind, solar, geothermal, and biomass power. These sources account for more than 35 percent of electric generation in Germany and more than 25 percent in California. Some proponents hope to reach total renewable energy by the end of the next decade.
In a recent book, A Bright Future, University of Massachusetts international relations scholar Joshua Goldstein and Staffan Qvist, a Swedish nuclear engineer, contend that renewable power cannot scale up quickly enough for rapid decarbonization, especially given those sources’ intermittency. Goldstein and Qvist argue that the current rate at which renewables are being deployed means that, rather than reaching total replacement of fossil fuels by 2030 or 2040, it might take over a century to decarbonize fully.
As an alternative, they point to Sweden: during a building spree in the late 1970s and early 1980s, the country built a fleet of nuclear reactors that (along with some large hydroelectric dams) produces the lion’s share of the nation’s electricity. In spite of consuming about one-third more electricity per capita than Germany, the Swedish electric grid is essentially carbon-free.
Only a crash program of nuclear plant building can avert catastrophe, Goldstein and Qvist say. However, the economics of replacing the 256 GW of coal power generating capacity in the United States (let alone elsewhere) with newly built nuclear power plants in just 10 years is daunting—especially at $6,000 per kW. And the questions of how to expand the nuclear supply chain or how to train a sufficient number of nuclear engineers in such short order remain unanswered.
Fortunately, the U.S. electrical system has been decarbonizing over the past decade without adding net nuclear capacity.
In a recent review, the U.S. Energy Information Administration reported annual U.S. carbon dioxide emissions from energy consumption in the electric power sector had fallen to 1,743 million metric tons (Mmt) in 2017, a drop of 32 percent from the 2005 level. This reduction of 673 Mmt occurred during a 13 year period when the total annual electrical net generation was close to level (3,902 billion kWh in 2005 and 3,878 billion kWh in 2017). This annual CO2 reduction is significant - almost equal to the total CO2 emissions of Germany (763.8 Mmt) in 2017!
How did this very significant U.S. CO2 reduction take place? Between 2005 and 2017, coal use was replaced by a 19 percent increase in the use of renewables (mostly wind) and a 31 percent increase in the use of natural gas.
Simply swapping fuels from coal to methane will reduce carbon dioxide emissions by half, given natural gas’s higher energy content per unit of carbon. And replacing the Rankine cycle coal plants with modern gas turbine combined-cycle plants nearly doubles the power plant thermal efficiency. Those two effects—reduced carbon density and increased thermal efficiency—combine so that switching from coal-fired steam turbine to a gas turbine combined-cycle plant will cut carbon emissions per unit of electricity by as much as 75 percent.
Since new use of natural gas in the U.S. means the addition of gas turbine electric plants, my assessment is that the record breaking CO2 reduction has occurred mostly by the use of dispatchable natural gas fueled gas turbines. As more combined cycle plants replace older coal plants we can expect additional significant reductions in CO2 emissions for the U.S., and elsewhere if others follow our lead.
At less than one-sixth the cost of nuclear!
Pathway to Zero Emissions
Currently, coal accounts for 30 percent of electrical production in the U.S. and 40 percent worldwide. Swapping that coal power with gas turbine combined-cycle plants will reduce CO2 emissions by 75 percent, but the ultimate goal is zero.
Gas turbines also can play a part in getting to zero, through a combination of old and new technology.
When the wind blows and the sun shines, wind turbines and solar devices produce electricity, sometimes more than can be consumed. That surplus renewable energy could be used to electrolyze water to produce hydrogen, a process that was discovered by William Nicholson and Anthony Carlisle in 1800. That electrolytic-produced hydrogen could either be stored or injected directly into natural gas pipeline systems. During lulls in solar and wind power production, the hydrogen would be tapped to fuel gas turbine combined-cycle plants.
Moving in two steps—first with methane-burning gas turbines and later converting those turbines to burn electrolyzed hydrogen—would enable a swift, affordable cut to carbon emissions in the near term and then allow a reduction to a zero-carbon system by repurposing those newly built plants. Such a plan ought to be far less expensive than building hundreds of new nuclear plants.
Just as the re-engining of the B-52s can keep them in the skies, serving an important defense role for decades to come, so too can “re-fueling” the gas turbine fleet with renewably produced hydrogen add years to existing assets, and new life to a now-stagnant market.
Lee. S. Langston is professor emeritus of mechanical engineering at the University of Connecticut in Storrs.
That plan hinges on swapping in commercial jet engines for the existing 17,000 pound-thrust TF-33 engines, which were designed in the late 1950s. As such, the airframes would be ancient by aviation standards, so much so that three generations of airmen may have served on the same bomber, but the engines would be state of the art. Does that make the planes old, or new?
Those TF-33s were designed a mere 20 years after the development of the first gas turbine in 1939. As prime movers go, gas turbines are virtually brand new, compared to, say, wind and water turbines which have been around for millennia. But they have also reached a considerable level of maturity. Gas turbines now dominate both the world’s aircraft propulsion and a good portion of electric power generation.
The fortunes of the industry are not uniform, however. The commercial jet engine market is robust and growing; the military jet engine, electric power, and other markets have been relatively flat or declining. But those are the sectors where the possibilities lie. They aren’t new, but they have the potential for renewal.
Aviation Dominates
Perhaps the best overview of the worldwide gas turbine market uses data from Forecast International, a market research firm in Newtown, Conn. Analyst Stuart Slade has tapped FI’s computer models and extensive database to calculate the value of gas turbine manufacturing production from 2009 to 2018, and has projected those values to 2033. (FI considers value of production figures to be more accurate than reported sales figures.)
Slade reports that the value of production for all gas turbines worldwide for 2018 was $86.5 billion, slightly down from $87.6 billion in 2017. As in years past, the largest portion of production values is in the aviation market -- $74.5 billion. Not only did the market grow at 3 percent from the 2017 level, but the value of production is forecast to grow to $92.2 billion by 2033.
Just as aviation dominates the total market, commercial jet engine manufacturing dominates aviation, with $66.1 billion in value of production in 2018. The production of jet engines follows the fortunes of its main customers, and the recent boom in commercial aviation has been so pronounced that over the past three years, the airline industry had generated more profit than the previous 30 years combined.
You May Also Like: Running in Place
Airlines have been clamoring for planes that use new, fuel-efficient engines, which has resulted in the popularity of the twin-engined Boeing 737 MAX and Airbus A320neo. To meet that demand, CFM International, a joint venture of GE Aviation and Safran Aircraft Engines, produced some 1,100 LEAP turbofan engine for such airliners last year, while Pratt & Whitney produced 750 of its PW 1000G series of geared turbofans. With more than 10,000 of those two airliners on order, both engine makers will be producing these engines for years to come.
The third major jet engine marker, Rolls-Royce, had some production issues in 2018 with their Trent 7000 engine, which at 70,000 lbt powers the Airbus A330neo, as well as durability problems with the Trent 1000 on Boeing’s 787. As a result, Rolls will not be bidding on an engine for Boeing’s New Midsize Airplane, the NMA, leaving the field to GE and Pratt & Whitney.
Narrow-body, single-aisle jets are the most profitable commercial aircraft, but the wide-body, twin-aisle airliner market still carries prestige. Those large planes are designed to cross oceans and need much larger engines than the 20,000-30,000 pound thrust LEAPs and PW 1000Gs. To give a sense of what the biggest planes require, General Electric will be flight testing their 100,000 lbt, GE9X Turbofan engine with an inlet fan diameter of 134 inches—just over 11 feet and nearly as wide as an A320 fuselage. The GE9X is intended to power Boeing’s new 777X, a twin-engine aircraft designed to carry more than 400 passengers.
In contrast to the boom in commercial jet engines, the other segment of the aviation market—jet engines for military aircraft—saw a slight decrease in value of production in 2018, to $8.4 billion. As noted above, some military airframes may start being re-engined with off-the-shelf commercial jets. But the specifications for military aircraft are so demanding that the technological advances needed to meet those specs filter down to both commercial jets and non-aviation gas turbines. Often, what’s revolutionary in those applications is old hat for military engines.
Most Efficient Heat Engines
The non-aviation market is not only considerably smaller than the aviation market, it’s more fragmented. Forecast International breaks out mechanical drive gas turbines, used extensively along natural gas pipelines to power compression stations, marine power gas turbines designed for ships, and microturbines are found in combined heat and power systems. The value of production for those three segments was $2.5 billion, compared to $9.5 billion for the fourth industry segment, gas turbines used in electric power generation.
The electric power segment has seen better days. In 2009, the total value of production was more than $20 billion, and it still more than $13 billion in 2017. Even looking out to 2033, Forecast International sees the value of production only partially recovering, reaching $12.3 billion.
The first gas turbine for use in electric generation was first tested 80 years ago at the Baden works of the Swiss company, Brown Boveri. Later it was installed at Neuchâtel, a city to the west of Baden. Burning fuel oil, it had a thermal efficiency of 18 percent and was used to power an electrical generator with an output up to 4 MW.
Read more about Unique Gas Turbine Engine Powering Quieter Drones
Today, simple-cycle gas turbines can reach efficiencies above 40 percent in units that generate as much as 500 MW. When matched with systems that use the high-temperature gas turbine exhaust to generate steam to run a Rankine-cycle turbine, combined-cycle gas turbines can reach thermal efficiencies as high as 60 percent—double that of most existing coal-fired plants—and power outputs greater than 1,000 MW. (As a mechanical engineer, I can’t resist repeatedly pointing out that gas turbines make up part of the most efficient heat engines yet perfected by mankind!)
These gas turbine combined-cycle (GTCC) plants also have low capital costs, in the range of $700 to $1,000 per kW, compared to $3,000 per kW for coal or $6,000 per kW for nuclear plants.
With record efficiency and low costs, GTCC plants were expected to be in high demand. But in 2018 both GE and Siemens, major gas turbine OEMs, cut their workforce significantly. It turned out that as larger and more efficient gas turbine plants have been installed, the need for more gas turbines was reduced. For various market reasons some gas turbine plants that were purchased in previous years have been mothballed, leading to a bow-wave of reserve capacity, limiting immediate demand.
Another factor leading to the recent downturn are market uncertainties caused by the introduction of increasingly cheap power from wind turbines and photovoltaic solar cells. Although these so-called renewable sources have depressed demand for new gas turbine power plants, over the long term, the intermittent nature of those facilities could increase the need for quick start gas turbine plants to back up the renewables when the sun doesn’t shine and the wind falters.
Crash Course
In an effort to decarbonize the production of electrical power, nations and large regions have added large amounts of wind, solar, geothermal, and biomass power. These sources account for more than 35 percent of electric generation in Germany and more than 25 percent in California. Some proponents hope to reach total renewable energy by the end of the next decade.
In a recent book, A Bright Future, University of Massachusetts international relations scholar Joshua Goldstein and Staffan Qvist, a Swedish nuclear engineer, contend that renewable power cannot scale up quickly enough for rapid decarbonization, especially given those sources’ intermittency. Goldstein and Qvist argue that the current rate at which renewables are being deployed means that, rather than reaching total replacement of fossil fuels by 2030 or 2040, it might take over a century to decarbonize fully.
As an alternative, they point to Sweden: during a building spree in the late 1970s and early 1980s, the country built a fleet of nuclear reactors that (along with some large hydroelectric dams) produces the lion’s share of the nation’s electricity. In spite of consuming about one-third more electricity per capita than Germany, the Swedish electric grid is essentially carbon-free.
Only a crash program of nuclear plant building can avert catastrophe, Goldstein and Qvist say. However, the economics of replacing the 256 GW of coal power generating capacity in the United States (let alone elsewhere) with newly built nuclear power plants in just 10 years is daunting—especially at $6,000 per kW. And the questions of how to expand the nuclear supply chain or how to train a sufficient number of nuclear engineers in such short order remain unanswered.
Fortunately, the U.S. electrical system has been decarbonizing over the past decade without adding net nuclear capacity.
In a recent review, the U.S. Energy Information Administration reported annual U.S. carbon dioxide emissions from energy consumption in the electric power sector had fallen to 1,743 million metric tons (Mmt) in 2017, a drop of 32 percent from the 2005 level. This reduction of 673 Mmt occurred during a 13 year period when the total annual electrical net generation was close to level (3,902 billion kWh in 2005 and 3,878 billion kWh in 2017). This annual CO2 reduction is significant - almost equal to the total CO2 emissions of Germany (763.8 Mmt) in 2017!
How did this very significant U.S. CO2 reduction take place? Between 2005 and 2017, coal use was replaced by a 19 percent increase in the use of renewables (mostly wind) and a 31 percent increase in the use of natural gas.
Simply swapping fuels from coal to methane will reduce carbon dioxide emissions by half, given natural gas’s higher energy content per unit of carbon. And replacing the Rankine cycle coal plants with modern gas turbine combined-cycle plants nearly doubles the power plant thermal efficiency. Those two effects—reduced carbon density and increased thermal efficiency—combine so that switching from coal-fired steam turbine to a gas turbine combined-cycle plant will cut carbon emissions per unit of electricity by as much as 75 percent.
Since new use of natural gas in the U.S. means the addition of gas turbine electric plants, my assessment is that the record breaking CO2 reduction has occurred mostly by the use of dispatchable natural gas fueled gas turbines. As more combined cycle plants replace older coal plants we can expect additional significant reductions in CO2 emissions for the U.S., and elsewhere if others follow our lead.
At less than one-sixth the cost of nuclear!
Pathway to Zero Emissions
Currently, coal accounts for 30 percent of electrical production in the U.S. and 40 percent worldwide. Swapping that coal power with gas turbine combined-cycle plants will reduce CO2 emissions by 75 percent, but the ultimate goal is zero.
Gas turbines also can play a part in getting to zero, through a combination of old and new technology.
When the wind blows and the sun shines, wind turbines and solar devices produce electricity, sometimes more than can be consumed. That surplus renewable energy could be used to electrolyze water to produce hydrogen, a process that was discovered by William Nicholson and Anthony Carlisle in 1800. That electrolytic-produced hydrogen could either be stored or injected directly into natural gas pipeline systems. During lulls in solar and wind power production, the hydrogen would be tapped to fuel gas turbine combined-cycle plants.
Moving in two steps—first with methane-burning gas turbines and later converting those turbines to burn electrolyzed hydrogen—would enable a swift, affordable cut to carbon emissions in the near term and then allow a reduction to a zero-carbon system by repurposing those newly built plants. Such a plan ought to be far less expensive than building hundreds of new nuclear plants.
Just as the re-engining of the B-52s can keep them in the skies, serving an important defense role for decades to come, so too can “re-fueling” the gas turbine fleet with renewably produced hydrogen add years to existing assets, and new life to a now-stagnant market.
Lee. S. Langston is professor emeritus of mechanical engineering at the University of Connecticut in Storrs.