A Whole New World
A Whole New World
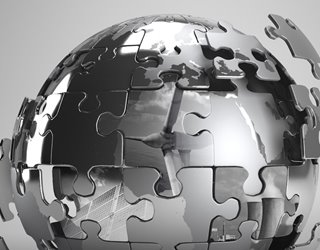
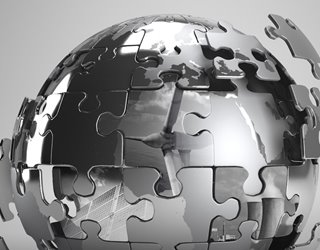
Switching to low-carbon energy systems could alter the global economic geography.
Winter camping in the Minnesota River valley is a remarkable experience. The wind that sculpts the snowdrifts largely disappears in the valley, so much so that when the temperature approaches 0 °F, the sound of person walking through the woods can be heard for half a mile or more. It is quieter than a sound-proof chamber.
One thing I recall vividly from a winter camping trip many years ago is effort needed to stay warm. For this, we needed to build and maintain a large fire. We cut up downed trees and hauled the wood back to the fire where the goal was to create not a blaze, but a thick bed of coals raked together by the time everyone went to bed. A layer of ash forms over the coals that keeps them insulated and hot during the night. Whoever got up in the early morning stirred the coals to get warm and added wood to quickly bring back the fire. The hot coals provided instant heat. While we did not think of it as a safety system, it was in fact a way to make winter camping safe against frostbite—enabled by stored heat of the hot coals and stored fuel in wood piles.
Camping is a choice, a recreational activity. But time in the frozen woods underscores the real challenges that faced humans in an era when they couldn’t rely on cheap, readily storable fossil fuels. Today, in mid-winter Minnesota, only about 2.5 percent of households rely on wood for home heating, while fully two-thirds of households use natural gas, pumped out of the ground in other U.S. states and Canada. Before the fossil fuel revolution of the past 150 years, however, survival in northern areas depended on manual labor and access to forests.
The labor devoted to the necessity of simply staying warm couldn’t be used for other purposes, and that fact had consequences that rippled outward. As difficult as it is to remember now, an era when “The Global North” is a metonym for the richest regions of the Earth, there was a time when to be northern was to be impoverished. In the middle ages, England was rich and Scandinavia was poor. England had good agricultural lands to feed people and animals with mild winters. Scandinavia had poor agricultural lands and severe winters with massive energy demands. If you lived in Scandinavia you spent much more time meeting your energy needs for food, heat, and light.
The arrival of fossil fuels changed everything. The fundamental economic attributes of fossils fuels—that they are cheap, easy to transport, and easy to store—made them a cornerstone commodity of the global trading system. A shipload of coal, oil, or liquefied natural gas costs about the same in New York Harbor as Shanghai, and one of the very largest supertankers can transport more than 2 million barrels of oil, or the energy equivalent of about half a million cords of wood.
Access to fossil fuels reshaped the world’s economic geography, fostering heavy industry close to coal beds and petrochemical plants near petroleum fields. The global automobile industry rises and falls on the worldwide price and availability of oil. The ability to tap fossil fuel reserves has enabled billions of people to reach the middle class.
Today, we live on the cusp of a transition away from fossil fuels. In order to eliminate carbon dioxide emissions implicated in global climate change, experts forecast that the world energy system will largely have to abandon coal and oil in the coming decades. It is unclear exactly what mix of new energy sources will replace fossil fuels: Will nuclear play a dominant role? Will solar and wind be deployed at unimaginable scale? But one little-appreciated aspect of this transition is that it will completely upend the world’s economic geography.
The world’s economic geography was different before fossil fuel age and it will be different after it.
For millennia, human society was limited in its power sources. In places with the right topology, people could exploit wind or water power; otherwise, the choices were animal traction, biomass, and human labor. As populations grew, forests were cleared to produce firewood for cooking and, in cold-weather locations, heating. Even as recently as 1900, Massachusetts was the least forested state in the United States because people had cut down its trees for fuel. With the demands of winter heating restraining growth in northern areas, it’s little wonder that the great cities of antiquity and the Middle Ages were generally found in warm-to-temperate weather areas: Mesopotamia, India, Central America, and the Mediterranean. (China was an exception to this rule.)
The widespread exploitation of coal beginning in the mid-19th century was a revolution. The switch from biomass to coal and then to oil resulted in old cities dying, new cities being built, and hundreds of millions of people moving worldwide. In Europe, the Ruhr valley of Germany, the Midlands of England, and the northeastern regions of France became industrial centers thanks to their ready access to coal. In the United States, the steel industry sprung up in Illinois, Indiana, Ohio, and Pennsylvania as iron ore from Minnesota was shipped down the Great Lakes to meet locally produced coal and limestone.
Spotlight on Nuclear Power: What Happened to the Nuclear Renaissance?
The advantages of fossil fuels extend to winter heating. Today, northern cities can be supplied with inexpensive heat due to the storability of fossil fuels. Heating oil is stored in tanks. Natural gas is moved year-round by pipeline into underground storage facilities near the customer; those storage facilities can store up to a quarter year’s production of natural gas. Natural gas for heating would be unaffordable if one had to build a long-distance pipeline system sized to meet peak heating demand.
The effects are more than economic. Thanks to home heating with fuel oil and natural gas, many of the forests of Massachusetts have been allowed to grow back since wood is now only a niche heating fuel.
All of this is being challenged by the prospects of a low- or zero-carbon energy system in response to climate change. All plans for curtailing carbon emissions call for coal and oil use to be phased out over the next 30 years or so. Some call for faster time frames and the elimination of natural gas, as well. These fuel sources, which today supply some 85 percent of the world’s energy, would have to be replaced at unprecedented speed with zero-net-carbon sources, such as nuclear, wind, solar, biomass, geothermal, and the like.
The economic impact of making such a rapid energy transition will be enormous, but the switch from fossil fuels to low-carbon sources will have similar geographical impacts. Energy is 8 percent of the gross domestic product, but for some industries the energy input is far greater. Large differences in the local cost of low-carbon energy services will cause mass movement of industry and jobs. Tens of millions of Americans and worldwide hundreds of millions of people will move in the transition to a low-carbon economy.
In a few places, a new industrial base may be built on wind or solar power. The northern coast of Chile, for instance, lies a short distance from the Atacama Desert, which is intensely dry, surrounded by high mountains, and near enough the Equator to receive intense sunlight 365 days a year. A low-cost solar industry in the Atacama, backed up by pumped hydro in mountain reservoirs, could power factories and electric-intensive aluminum smelters on the coast.
Texas may also be a big winner, its historic association with the oil industry notwithstanding. The state features summer peak electricity demands, low-cost wind, low-cost solar, cheap natural gas, and the geology to support cheap carbon-dioxide sequestration.
However, places like that are few and far between.
The challenge of the low-carbon transition is particularly apparent in the electric sector.
Electricity grew up with fossil fuels, and the sector has been shaped by the economics of fossil-fuel combustion. (This is an accident of history; wind and water power long predated the utilization of fossil fuels, and it’s possible to imagine an alternative electricity sector built around the strengths and weaknesses of those sources.) The capital cost of a fossil-fuel plant is less than the cost of the fossil fuels burnt in that plant. That means the cost of running a plant varies in sync with the plant’s output. As a consequence it is economic to operate fossil plants at part load to match electricity demand on an hourly to seasonal basis.
More by This Author: Storing Heat from Nuclear Power Plants Could Improve Output
In a low-carbon world of nuclear, wind, and solar power, providing dispatchable electricity becomes a much larger logistical and economic challenge. Each of these technologies has high capital costs and low operating costs, which means that the cost of operating the facility is roughly the same whether it is producing at full capacity or at a fraction. Thus, operate any of these technologies at half capacity and the cost of energy doubles.
If you compare the smoothed electricity demand for California over a period of one year to the smoothed output of wind and solar facilities of a scale sufficient to match the total electricity demand, you’ll see a seasonal mismatch between production and demand. The mismatch between electricity demand and the solar/wind output explains why one sees large increases in electricity prices in Germany, California, and other places where wind and solar have been added at a large scale. Because of that mismatch, one needs a second set of power generators to produce electricity at times of low wind and solar, with associated costs of essentially two electric generating systems. The second system provides assured electric generating capacity while wind and solar primarily operate in a fuel saving mode. (Nuclear power with a flat production provides a closer match for the seasonal effects.)
Storage technologies such as batteries and even pumped hydro storage do not address seasonal mismatches between production and demand; thus, they do not address the major challenge for a low-carbon grid.
The challenge of a low- to zero-carbon energy system is compounded by the issue of heating. Although virtually all the coal demand in the United States today comes from the electric power and steel sectors, some 65 percent of natural gas goes to residential, commercial, or industrial customers, with a large fraction of that used for process or space heating. Indeed, the total industrial heat demand (including input from oil and biomass as well as gas) is twice the total electricity output of the United States. If we decarbonize the economy, electricity will replace much of the heating demand met by fossil fuels.
While demand for industrial process heat is more or less constant, commercial and residential heat demand varies with location and season, as do wind and solar resources, but they do not generally vary in sync with one another. With heating needs met by electricity rather than gas or fuel oil, peak electricity demand will be in the winter, a time of minimal solar and little wind.
While heat pumps can meet some of the residential and commercial heating load and lower electricity demand, they become non-functional near the freezing point where resistance heating becomes the norm. Technical solutions such as ground-source heat pumps and large-scale heat storage take space and are not viable in towns or cities with higher population densities. Super-insulated buildings imply replacing much of the housing in parts of the United States.
If none of those options are feasible, then additional renewable electricity capacity will have to be built to meet the seasonable heating demand. Not only is electricity more expensive—by about a factor of six at today’s prices—but since solar output in particular is reduced in the regions with the largest winter heating demand, long-distance transmission lines will have to be built. Adding to the cost, those transmission lines would have to be sized for the wintertime peak that would occur for only a limited number of hours per year.
The additional cost and sometimes limited availability of renewable energy will have a negative impact on some regions. The increased price for process heating has the potential to make much of U.S. industry non-competitive. Whole industries defined by fossil fuel sources will move. In a low-carbon world, for instance, the steel industry’s historic base in coal-rich regions will shift to follow energy availability. Iron ore may be converted to steel using hydrogen made from wind and nuclear power in Minnesota, since there is no need to move the iron ore to the fossil fuel. An alternative would enable more traditional fossil processes by building steel mills where carbon sequestration sites allow low net-carbon steel to be produced.
If these scenarios seem vague, that is because the fossil-fuel system is so deeply embedded into our industrial system that we have only a limited understanding of what happens when the fossil fuels disappear.
Climate Solutions: Building the Ultimate Carbon Capture Tree
The shift away from cheap storable fossil fuels for heating will also likely drive up the cost of living dramatically in places such as New England—just like it did for Scandinavia in the Middle Ages. Indeed, in a low-carbon world, one can expect that New England will become the poorest part of the contiguous United States because of the high cost of energy services.
Geographical energy poverty will only be limited by nuclear energy because it has the storability and transportability of fossil fuels; that is, it is not dependent upon the local climate and thus not dependent upon location. The degree of disruption in going to a low-carbon economy will partly depend upon the cost of nuclear power and societal decisions. Compared to an all-renewable energy system, the cost of electricity with a substantial nuclear component cuts the cost of electricity in New England by half—reflecting the poor match between renewables electricity production and electricity demand curves. The impact is smaller in Texas, where the peak electric demand from air conditioning better matches renewables production. The differential in cost impact also implies large variations in choice of generating technology compared to today.
Another change that seems obvious is that in places where nuclear power is available, it will be used to address industrial process heating demand. In spite of the close association with the electric power industry, nuclear reactors produce heat much more cheaply than electricity. It takes several units of heat to produce one unit of electricity thanks to the laws of thermodynamics. (In contrast, wind and solar photovoltaic produce electricity where one unit of electricity produces one unit of heat.) That implies heat from a nuclear reactor costs a third of the price of electricity.
Nuclear cogeneration—where heat from nuclear reactors provide heat to industry coupled with electricity production and low-cost gigawatt-hour heat storage—should become widespread. It would build on existing models: Fossil-fuel cogeneration is used in the chemical industry, and there are a few sites where industrial cogeneration plants provide electricity and heat to multiple local industries. Cogeneration enables high-temperature steam to be used for electricity production with the lower-temperature steam from high-pressure turbines used in industrial production, wringing two uses out of the same unit of heat. It enables the optimization of the combined electric and industrial energy demand to minimize total costs.
While large heat users could be expected to have their own reactors, smaller users likely would find it necessary to move into large industrial parks with common cogeneration plants for many customers. The industrial infrastructure will change so that the nuclear reactors (or fossil plants with carbon dioxide sequestration) provide high-temperature heat in the form of steam, hot heat-transfer oil, or hot salt to multiple nearby industrial plants—industrial parks built around a central heat source.
In the future, then, the optimal places to site factories and facilities may be radically different from what is considered the best places today. In a low-carbon world, regions that efficiently deploy nuclear energy for heat will have a competitive advantage in becoming industrial superpowers—as will locations such as Texas, with the combination of cheap natural gas and carbon dioxide sequestration sites, and locations blessed with a combination of a reliable renewable power supply and the geography to support pumped hydro storage. The current model of dispersed smaller industrial facilities with natural gas piped in, heat applied to industrial processes, and carbon dioxide dumped into the atmosphere may largely disappear.
When I think of the energy transition of the past, I think back to river valley woodlands where I went camping so many winters ago, or of the newly reforested Massachusetts landscape. In a low-carbon world where heating is suddenly expensive again, those trees would all be gone, cut down for firewood.
Every previous energy transition has resulted in massive changes to society, mass movement of industry and people, and large changes in the relative standards of living of different parts of the world. Cheap coal provided the industrial might to build the British Empire, but places built around watermills lost their competitive advantages. Widely available oil helped cars and trucks reshape the landscape, but railroad towns faded. Today, cheap natural gas is responsible for much of the rebuilding of American industry—we have the lowest price natural gas in the world—but coal mining regions are dying.
Editor’s Choice: What Do You Know About Small Nuclear Reactors?
There is no reason to believe that moving to a low-carbon energy system will be different. There will be geographic winners and losers.
Going to a low-carbon world partly wipes the slate clean for different countries to become the industrial superpowers of the 21st century. The countries that successfully integrate nuclear, wind, and solar in such a way to minimize total energy costs will thrive.
The way to preserve the vitality of the today’s industrial heartlands is to find renewable and nuclear energy solutions to match the cost, storability, and transportability of fossil fuels. For the Industrial Midwest of the United States, the Rhine-Ruhr Valleys of Germany, or the manufacturing centers of eastern China, that is the engineering challenge of the 21st century. Failing that, we will have a world where location determines which places enjoy high standards of living, which countries are the new industrial superpowers, and which are impoverished—just as in the Middle Ages.
Charles W. Forsberg is a principal research scientist in the Department Nuclear Science and Engineering at the Massachusetts Institute of Technology in Cambridge and principle investigator of the DOE Integrated Research Project on Fluoride-Salt-Cooled High-Temperature Reactors.
One thing I recall vividly from a winter camping trip many years ago is effort needed to stay warm. For this, we needed to build and maintain a large fire. We cut up downed trees and hauled the wood back to the fire where the goal was to create not a blaze, but a thick bed of coals raked together by the time everyone went to bed. A layer of ash forms over the coals that keeps them insulated and hot during the night. Whoever got up in the early morning stirred the coals to get warm and added wood to quickly bring back the fire. The hot coals provided instant heat. While we did not think of it as a safety system, it was in fact a way to make winter camping safe against frostbite—enabled by stored heat of the hot coals and stored fuel in wood piles.
Camping is a choice, a recreational activity. But time in the frozen woods underscores the real challenges that faced humans in an era when they couldn’t rely on cheap, readily storable fossil fuels. Today, in mid-winter Minnesota, only about 2.5 percent of households rely on wood for home heating, while fully two-thirds of households use natural gas, pumped out of the ground in other U.S. states and Canada. Before the fossil fuel revolution of the past 150 years, however, survival in northern areas depended on manual labor and access to forests.
The labor devoted to the necessity of simply staying warm couldn’t be used for other purposes, and that fact had consequences that rippled outward. As difficult as it is to remember now, an era when “The Global North” is a metonym for the richest regions of the Earth, there was a time when to be northern was to be impoverished. In the middle ages, England was rich and Scandinavia was poor. England had good agricultural lands to feed people and animals with mild winters. Scandinavia had poor agricultural lands and severe winters with massive energy demands. If you lived in Scandinavia you spent much more time meeting your energy needs for food, heat, and light.
The arrival of fossil fuels changed everything. The fundamental economic attributes of fossils fuels—that they are cheap, easy to transport, and easy to store—made them a cornerstone commodity of the global trading system. A shipload of coal, oil, or liquefied natural gas costs about the same in New York Harbor as Shanghai, and one of the very largest supertankers can transport more than 2 million barrels of oil, or the energy equivalent of about half a million cords of wood.
Access to fossil fuels reshaped the world’s economic geography, fostering heavy industry close to coal beds and petrochemical plants near petroleum fields. The global automobile industry rises and falls on the worldwide price and availability of oil. The ability to tap fossil fuel reserves has enabled billions of people to reach the middle class.
Today, we live on the cusp of a transition away from fossil fuels. In order to eliminate carbon dioxide emissions implicated in global climate change, experts forecast that the world energy system will largely have to abandon coal and oil in the coming decades. It is unclear exactly what mix of new energy sources will replace fossil fuels: Will nuclear play a dominant role? Will solar and wind be deployed at unimaginable scale? But one little-appreciated aspect of this transition is that it will completely upend the world’s economic geography.
The world’s economic geography was different before fossil fuel age and it will be different after it.
Industrial Heartlands
For millennia, human society was limited in its power sources. In places with the right topology, people could exploit wind or water power; otherwise, the choices were animal traction, biomass, and human labor. As populations grew, forests were cleared to produce firewood for cooking and, in cold-weather locations, heating. Even as recently as 1900, Massachusetts was the least forested state in the United States because people had cut down its trees for fuel. With the demands of winter heating restraining growth in northern areas, it’s little wonder that the great cities of antiquity and the Middle Ages were generally found in warm-to-temperate weather areas: Mesopotamia, India, Central America, and the Mediterranean. (China was an exception to this rule.)
The widespread exploitation of coal beginning in the mid-19th century was a revolution. The switch from biomass to coal and then to oil resulted in old cities dying, new cities being built, and hundreds of millions of people moving worldwide. In Europe, the Ruhr valley of Germany, the Midlands of England, and the northeastern regions of France became industrial centers thanks to their ready access to coal. In the United States, the steel industry sprung up in Illinois, Indiana, Ohio, and Pennsylvania as iron ore from Minnesota was shipped down the Great Lakes to meet locally produced coal and limestone.
Spotlight on Nuclear Power: What Happened to the Nuclear Renaissance?
The advantages of fossil fuels extend to winter heating. Today, northern cities can be supplied with inexpensive heat due to the storability of fossil fuels. Heating oil is stored in tanks. Natural gas is moved year-round by pipeline into underground storage facilities near the customer; those storage facilities can store up to a quarter year’s production of natural gas. Natural gas for heating would be unaffordable if one had to build a long-distance pipeline system sized to meet peak heating demand.
The effects are more than economic. Thanks to home heating with fuel oil and natural gas, many of the forests of Massachusetts have been allowed to grow back since wood is now only a niche heating fuel.
All of this is being challenged by the prospects of a low- or zero-carbon energy system in response to climate change. All plans for curtailing carbon emissions call for coal and oil use to be phased out over the next 30 years or so. Some call for faster time frames and the elimination of natural gas, as well. These fuel sources, which today supply some 85 percent of the world’s energy, would have to be replaced at unprecedented speed with zero-net-carbon sources, such as nuclear, wind, solar, biomass, geothermal, and the like.
The economic impact of making such a rapid energy transition will be enormous, but the switch from fossil fuels to low-carbon sources will have similar geographical impacts. Energy is 8 percent of the gross domestic product, but for some industries the energy input is far greater. Large differences in the local cost of low-carbon energy services will cause mass movement of industry and jobs. Tens of millions of Americans and worldwide hundreds of millions of people will move in the transition to a low-carbon economy.
In a few places, a new industrial base may be built on wind or solar power. The northern coast of Chile, for instance, lies a short distance from the Atacama Desert, which is intensely dry, surrounded by high mountains, and near enough the Equator to receive intense sunlight 365 days a year. A low-cost solar industry in the Atacama, backed up by pumped hydro in mountain reservoirs, could power factories and electric-intensive aluminum smelters on the coast.
Texas may also be a big winner, its historic association with the oil industry notwithstanding. The state features summer peak electricity demands, low-cost wind, low-cost solar, cheap natural gas, and the geology to support cheap carbon-dioxide sequestration.
However, places like that are few and far between.
Logistical Challenges
The challenge of the low-carbon transition is particularly apparent in the electric sector.
Electricity grew up with fossil fuels, and the sector has been shaped by the economics of fossil-fuel combustion. (This is an accident of history; wind and water power long predated the utilization of fossil fuels, and it’s possible to imagine an alternative electricity sector built around the strengths and weaknesses of those sources.) The capital cost of a fossil-fuel plant is less than the cost of the fossil fuels burnt in that plant. That means the cost of running a plant varies in sync with the plant’s output. As a consequence it is economic to operate fossil plants at part load to match electricity demand on an hourly to seasonal basis.
More by This Author: Storing Heat from Nuclear Power Plants Could Improve Output
In a low-carbon world of nuclear, wind, and solar power, providing dispatchable electricity becomes a much larger logistical and economic challenge. Each of these technologies has high capital costs and low operating costs, which means that the cost of operating the facility is roughly the same whether it is producing at full capacity or at a fraction. Thus, operate any of these technologies at half capacity and the cost of energy doubles.
If you compare the smoothed electricity demand for California over a period of one year to the smoothed output of wind and solar facilities of a scale sufficient to match the total electricity demand, you’ll see a seasonal mismatch between production and demand. The mismatch between electricity demand and the solar/wind output explains why one sees large increases in electricity prices in Germany, California, and other places where wind and solar have been added at a large scale. Because of that mismatch, one needs a second set of power generators to produce electricity at times of low wind and solar, with associated costs of essentially two electric generating systems. The second system provides assured electric generating capacity while wind and solar primarily operate in a fuel saving mode. (Nuclear power with a flat production provides a closer match for the seasonal effects.)
Storage technologies such as batteries and even pumped hydro storage do not address seasonal mismatches between production and demand; thus, they do not address the major challenge for a low-carbon grid.
The challenge of a low- to zero-carbon energy system is compounded by the issue of heating. Although virtually all the coal demand in the United States today comes from the electric power and steel sectors, some 65 percent of natural gas goes to residential, commercial, or industrial customers, with a large fraction of that used for process or space heating. Indeed, the total industrial heat demand (including input from oil and biomass as well as gas) is twice the total electricity output of the United States. If we decarbonize the economy, electricity will replace much of the heating demand met by fossil fuels.
While demand for industrial process heat is more or less constant, commercial and residential heat demand varies with location and season, as do wind and solar resources, but they do not generally vary in sync with one another. With heating needs met by electricity rather than gas or fuel oil, peak electricity demand will be in the winter, a time of minimal solar and little wind.
While heat pumps can meet some of the residential and commercial heating load and lower electricity demand, they become non-functional near the freezing point where resistance heating becomes the norm. Technical solutions such as ground-source heat pumps and large-scale heat storage take space and are not viable in towns or cities with higher population densities. Super-insulated buildings imply replacing much of the housing in parts of the United States.
If none of those options are feasible, then additional renewable electricity capacity will have to be built to meet the seasonable heating demand. Not only is electricity more expensive—by about a factor of six at today’s prices—but since solar output in particular is reduced in the regions with the largest winter heating demand, long-distance transmission lines will have to be built. Adding to the cost, those transmission lines would have to be sized for the wintertime peak that would occur for only a limited number of hours per year.
Geographical Energy Poverty
The additional cost and sometimes limited availability of renewable energy will have a negative impact on some regions. The increased price for process heating has the potential to make much of U.S. industry non-competitive. Whole industries defined by fossil fuel sources will move. In a low-carbon world, for instance, the steel industry’s historic base in coal-rich regions will shift to follow energy availability. Iron ore may be converted to steel using hydrogen made from wind and nuclear power in Minnesota, since there is no need to move the iron ore to the fossil fuel. An alternative would enable more traditional fossil processes by building steel mills where carbon sequestration sites allow low net-carbon steel to be produced.
If these scenarios seem vague, that is because the fossil-fuel system is so deeply embedded into our industrial system that we have only a limited understanding of what happens when the fossil fuels disappear.
Climate Solutions: Building the Ultimate Carbon Capture Tree
The shift away from cheap storable fossil fuels for heating will also likely drive up the cost of living dramatically in places such as New England—just like it did for Scandinavia in the Middle Ages. Indeed, in a low-carbon world, one can expect that New England will become the poorest part of the contiguous United States because of the high cost of energy services.
Geographical energy poverty will only be limited by nuclear energy because it has the storability and transportability of fossil fuels; that is, it is not dependent upon the local climate and thus not dependent upon location. The degree of disruption in going to a low-carbon economy will partly depend upon the cost of nuclear power and societal decisions. Compared to an all-renewable energy system, the cost of electricity with a substantial nuclear component cuts the cost of electricity in New England by half—reflecting the poor match between renewables electricity production and electricity demand curves. The impact is smaller in Texas, where the peak electric demand from air conditioning better matches renewables production. The differential in cost impact also implies large variations in choice of generating technology compared to today.
Another change that seems obvious is that in places where nuclear power is available, it will be used to address industrial process heating demand. In spite of the close association with the electric power industry, nuclear reactors produce heat much more cheaply than electricity. It takes several units of heat to produce one unit of electricity thanks to the laws of thermodynamics. (In contrast, wind and solar photovoltaic produce electricity where one unit of electricity produces one unit of heat.) That implies heat from a nuclear reactor costs a third of the price of electricity.
Nuclear cogeneration—where heat from nuclear reactors provide heat to industry coupled with electricity production and low-cost gigawatt-hour heat storage—should become widespread. It would build on existing models: Fossil-fuel cogeneration is used in the chemical industry, and there are a few sites where industrial cogeneration plants provide electricity and heat to multiple local industries. Cogeneration enables high-temperature steam to be used for electricity production with the lower-temperature steam from high-pressure turbines used in industrial production, wringing two uses out of the same unit of heat. It enables the optimization of the combined electric and industrial energy demand to minimize total costs.
While large heat users could be expected to have their own reactors, smaller users likely would find it necessary to move into large industrial parks with common cogeneration plants for many customers. The industrial infrastructure will change so that the nuclear reactors (or fossil plants with carbon dioxide sequestration) provide high-temperature heat in the form of steam, hot heat-transfer oil, or hot salt to multiple nearby industrial plants—industrial parks built around a central heat source.
In the future, then, the optimal places to site factories and facilities may be radically different from what is considered the best places today. In a low-carbon world, regions that efficiently deploy nuclear energy for heat will have a competitive advantage in becoming industrial superpowers—as will locations such as Texas, with the combination of cheap natural gas and carbon dioxide sequestration sites, and locations blessed with a combination of a reliable renewable power supply and the geography to support pumped hydro storage. The current model of dispersed smaller industrial facilities with natural gas piped in, heat applied to industrial processes, and carbon dioxide dumped into the atmosphere may largely disappear.
Making the Transition
When I think of the energy transition of the past, I think back to river valley woodlands where I went camping so many winters ago, or of the newly reforested Massachusetts landscape. In a low-carbon world where heating is suddenly expensive again, those trees would all be gone, cut down for firewood.
Every previous energy transition has resulted in massive changes to society, mass movement of industry and people, and large changes in the relative standards of living of different parts of the world. Cheap coal provided the industrial might to build the British Empire, but places built around watermills lost their competitive advantages. Widely available oil helped cars and trucks reshape the landscape, but railroad towns faded. Today, cheap natural gas is responsible for much of the rebuilding of American industry—we have the lowest price natural gas in the world—but coal mining regions are dying.
Editor’s Choice: What Do You Know About Small Nuclear Reactors?
There is no reason to believe that moving to a low-carbon energy system will be different. There will be geographic winners and losers.
Going to a low-carbon world partly wipes the slate clean for different countries to become the industrial superpowers of the 21st century. The countries that successfully integrate nuclear, wind, and solar in such a way to minimize total energy costs will thrive.
The way to preserve the vitality of the today’s industrial heartlands is to find renewable and nuclear energy solutions to match the cost, storability, and transportability of fossil fuels. For the Industrial Midwest of the United States, the Rhine-Ruhr Valleys of Germany, or the manufacturing centers of eastern China, that is the engineering challenge of the 21st century. Failing that, we will have a world where location determines which places enjoy high standards of living, which countries are the new industrial superpowers, and which are impoverished—just as in the Middle Ages.
Charles W. Forsberg is a principal research scientist in the Department Nuclear Science and Engineering at the Massachusetts Institute of Technology in Cambridge and principle investigator of the DOE Integrated Research Project on Fluoride-Salt-Cooled High-Temperature Reactors.
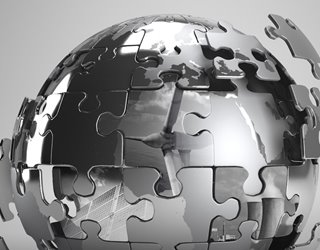
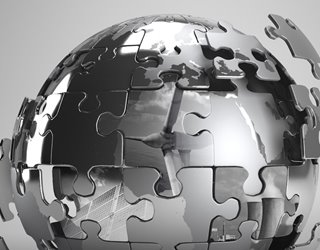