What Termites Can Teach Engineers
What Termites Can Teach Engineers
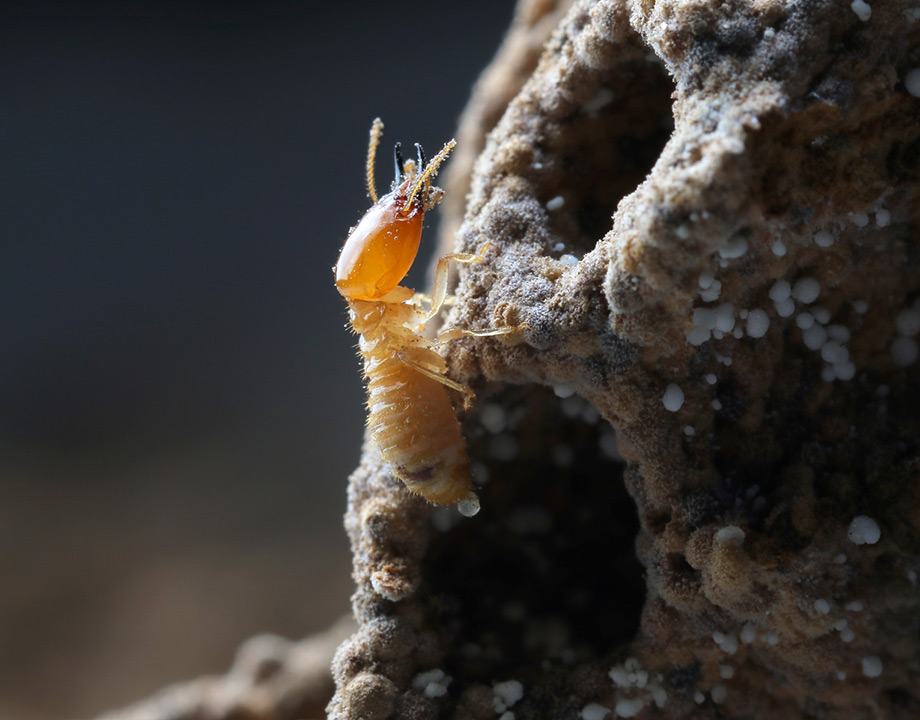
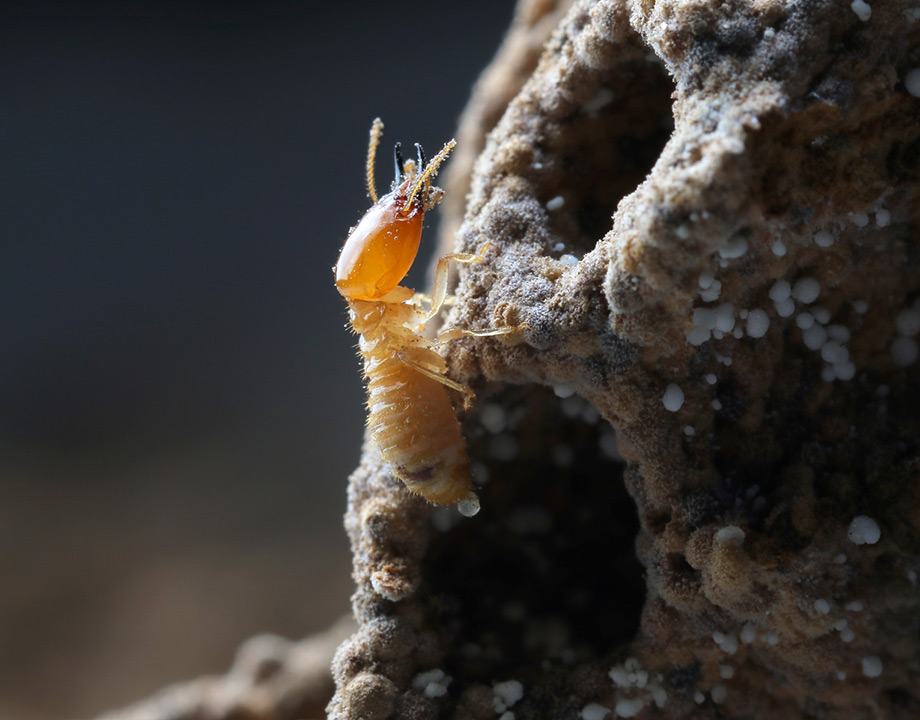
Termite may have brains the size of a grain of sand, but they can school engineers and architects about heating and cooling. Photo: Getty
We humans like to congratulate ourselves for our ingenuity. Yet nature’s passive designs often outperform our expensive, energy-hungry technologies. And while engineers and architects can improve their designs by mimicking the natural world, nature always has another lesson to teach. That has certainly been the case for termites and air conditioning.
The story begins in 1992, when Zimbabwean architect Mick Pearce received a commission to build Eastgate Centre, a two-building office complex and shopping mall, in the country’s capital city of Harare. Pearce, however, wanted to do more than just build a new building. He wanted to eliminate the huge heating and cooling plants a 340,000 square feet development typically needed.
Pearce already had a solution in mind. He began to formulate it while watching BBC’s natural history series, Life, on TV one evening.
“I saw David Attenborough climbing inside the chimney of a termite nest in Nigeria,” he said, and he realized that evolution had already solved the problem.
Pearce had grown up in Zimbabwe and was familiar with such chimneys. They rose six to 30 feet above termite nests and were strong enough for elephants to use to scratch their flanks.
Below the chimneys, the termites farmed fungi for food. While the termites relied on soil’s thermal storage capacity to help keep temperatures stable, Pearce realized that the termites also had to breathe.
It was an elegant design. The hot air generated by the nest and its fungus farm, which had higher concentrations of carbon dioxide and methane, exited through the chimney through convention. As the hot air left, it pulled in fresh air from the surface through moist foraging tunnels, which added water vapor to the stream. The chimney, warmed by the sun, heated the air exiting through it, adding an extra push to the convection cycle.
“The termite nest was warmer than the outside, particularly at night,” Pearce said in an interview. “You get air rising out the chimney, and that pulls air in from the holes they go out to forage. That’s how they breathe.”
If termites could do it, Pearce thought, why not humans?
Pearce had one thing going for him: Although Zimbabwe is semitropical, Harare’s 4800 ft. elevation keeps it cool and provides an average temperature swing of about 10 degrees Celsius each day. Pearce figured he could pull in night air to cool concrete and masonry structures. They would act like the soil in a termite nest to store cooling. He could then chill incoming air by running it over those structures during the day.
In the building that Pearce and multidisciplinary engineering firm Arup Group designed, each floor had air ducts running underneath it. These ducts contained concrete blocks with protruding teeth, whose high surface area enabled them to cool down and heat up efficiently.
Banks of fans drove the cool air through the shopping area and seven floors of offices. As the air warmed, it rose and exited through a central atrium connecting the two towers and 48 brick chimneys that ran along their roofs.
Pearce’s designed also used several other tricks inspired by nature. Office windows, for example, are shaded by overhangs and plants to keep sunlight from heating the building too quickly. The exterior features jagged gabbles and facades that act like cactus spikes, whose high surface areas disperse heat rapidly at night.
On many levels, worked spectacularly well. Eastgate cost 10 percent less to build than a similarly sized building with air conditioning. The building also used 35 percent energy when comparable buildings in Harare and saved $3.5 million in energy costs during its first five years.
Further Reading: Storing Heat from Nuclear Power Plants Could Improve Output
Of course, office temperatures are not as tightly controlled as conventional buildings. Most of the time, they hover in the mid-70s degrees Fahrenheit range, but they may reach 80-82 degrees at the end of the day for several weeks each year. This led Pearce and Ove Arup to improve the control system.
“The trick is to coordinate the weather outside with the controlled internal environment, taking into account lag times,” he said. “It’s like tuning an instrument, like an organ in a church.”
Pearce was a pioneer of biomimicry, which draws inspiration from the natural world for human design. He went on to apply his approach to a more efficient office block in Melbourne, Australia, and build a tower and dining hall in China.
“I was convinced that by looking at animal architecture, there are important clues for an architecture which inspires the design team to make buildings that follow natural processes, cycles, and systems,” he said.
Yet despite his successes, Pearce did not learn everything termites had to teach him.
Since Eastgate, scientists have learned much more about how mound-building termites (and other animals) work their architectural magic. This has led to a new approach that physiologist J. Scott Turner and architect Rupert C. Soar call “beyond biomimicry.” They want to create “buildings that are not simply inspired by life,” they wrote in a 2008 paper, “but that are, in a sense, as alive as their inhabitants.”
New research techniques that can reveal the unseen intricacies of natural structures makes this goal possible.
One example involves the use of 3D X-ray tomographic imaging. Kamaljit Singh of the Department of Earth Science at Imperial College London and his colleagues used the technique to image sections of termite nests from Senegal and Guinea. This provided unprecedented details of the nest wall microstructure.
While both nests were built by the same species, Trinervitermes geminatus, they used very different construction materials, depending on the local environment. Yet both sets of walls showed similar—and surprising—features.
All the walls contained an intricate network of micropores, with smaller pores deep inside the nest transitioning to larger pores near on outer walls. This network facilitates the exchange of carbon dioxide with the outside and drain rainwater from the nest by capillary action.
When the researchers used their 3D tomographic images to simulate these processes, they found they operated much like gaseous diffusion within human lungs.
The work demonstrates that Pearce’s Eastgate design, as successful as it is, got it wrong.
You May Also Like: Origami Robots Move Like Ants and Work Like Ants
“Eastgate Centre was built on a wrong conception of how termites regulate the temperature of their nests,” Singh’s co-author, Guy Theraulaz of the University of Toulouse, said. “Still, Eastgate works.”
“It is appropriate to speak of [Eastgate] as ‘termite-inspired’ design,” Turner, a biologist at the SUNY College of Environmental Science and Forestry, added. “The architect, Mick Pearce, drew more on inspiration rather than knowledge of how these mounds worked.
“In the end, he created a successful building design because he approached the engineering challenge—a building in semi-tropical climate without an air conditioning plant—in an imaginative way.
“What made this design successful was several ‘termite-inspired’ ideas, like tall stacks, porous walls, and a large central atrium that could draw air passively through the building. What is often not mentioned is the clever use of large heat storage blocks that could damp temperatures through the hot day, while using high-powered fans to ‘empty’ those heat stores during the cool night. Termites do not have the latter. Termites do not use this to maintain temperature.”
So if the Eastgate model isn’t quite right, what is?
Scientists are discovering that termite heating and cooling is far more subtle and complex—especially because different termite species use different techniques, depending on their individual environments and traits.
The species that inspired Mick Pearce and his Eastgate Centre design farms fungus, and the termites must remove the carbon dioxide the fungus generates so they do not suffocate. That problem is solved by the large chimney structures.
Other species, such as the one studied by Singh, build closed nests with no ventilation to the outside world. Instead of chimneys, they use the micropore structure to let the nest “breathe” like a lung.
While it’s been known for some time that termites had developed some sort of mechanisms for controlling the internal environment of their nests, researchers disagreed on the details.
Termites obviously used convective process to circulate heat and air, but what drove it? Did the wind create differences in air pressure between the nest interior and exterior, inducing a Venturi flow of air? Was it an internal metabolic process?
It was challenging to pin down the mechanism without complete or direct measurements. This made it necessary to infer interior temperatures, air flow rates, CO2 concentrations, and other variables. The differences between termite species made it all more complicated.
Harvard physicist and mathematician L. Mahadevan and his colleagues, including Turner, Soar, and Samuel Ocko, have tried to cut through the confusion to find some broader principles that held across various termite species.
First, they needed better data. Mahadevan put together an interdisciplinary team, which developed a custom sensor to measure airflow within the mounds themselves. In 2017, they conducted more than one hundred measurements daily in 30 mounds of the African species Macrotermes michaelseni to get a 24-hour picture. They also measured external winds as well as CO2 and temperature in various parts of the mounds.
The team then compared the results with similar measurements they made on Asian termites in 2015. Mahadevan, Ocko, and Alexander Heyde put it all together in a mathematical model that they published in 2019.
The lung analogy turns out to be even more apt than previously thought. The mounds “breathe” both out and in, as the temperatures vary over the course of a 24-hour period.
“One needs to have variations in diurnal temperatures that can drive circulation because of temperature gradients that switch between day and night,” Mahadevan explains.
Recognizing the role of temperature was hardly a new insight, he said. “The innovation was in thinking about the problem differently—by recognizing that diurnal variations can drive flows that ventilate the mound.”
As the heating sunlight moves across the surface of the mound in the course of the day, different parts warm up and then cool. This shifts the temperature gradients inside the mound, which drives the internal airflow. Instead of relying on wind blowing over the mound, termites manage airflow within the nest with something even simpler and more predictable: sunlight. In a real sense, these insects, or at least their climate control, are solar powered.
According to Mahadevan, these daily temperature oscillations drive convective flow, which reverses twice a day and thus serves to ventilate the mound. The study showed that neither wind (of which there was little to be found locally) nor termite metabolism played a role in ventilation, contrary to prior hypotheses.
The same principles should hold across the termite universe, for the fungus-farming chimney builders as well as their less agriculturally inclined, closed-mound living relatives.
“Because daily oscillations in radiative heating are a fact of life on our planet, this mechanism is likely to be generic across different species of mound-building termites,” Mahadevan wrote in his 2017 study. In fact, the researchers speculated that such other animals might take advantage of solar heating to control their ventilation as well.
Often, termites actively manage this process, blocking and opening new tunnels to modify airflow.
That works for termites, but what about human beings? Mahadevan believes there’s no reason that architects could not apply the same principles to their buildings.
Recommended for You: Bots and Bugs in Harmony
“This ought to work in buildings once we recognize that differential heating of the center and the periphery suffice to drive flow,” he said.
Unfortunately, what ought to work in theory does not always pan out in the real world.
Instead, “the mechanism that you find at the scale of the termites has to be adapted,” Theraulaz said. “So we have to discover new principles to design the architecture to get the same kind of properties. When we understand what happens at the microscale, we can build models and try to see what kind of architecture we can build.”
New technologies, such as Mahadevan’s sensors and simulations, promise that easier.
“We now have a set of techniques by which we are able to analyze nest architectures,” Theraulaz said. “In the past 10 years, we have scanned more than one hundred termite nests, so we have a huge basis [for analyzing their] architecture.
“We can apply the same [simulation] techniques to achieve a broader understanding of how these structures play a role in thermoregulation. For the moment, we don’t have a clear idea. Perhaps there are multiple ways to achieve the same kind of thing. It’s something we are beginning to explore.
“The most interesting thing is that we can apply the same tools we use to analyze the nest architecture to human architecture, to analyze the diffusion of gases, how temperature behaves inside. And by simulation we can try to find the parameters in the architecture that will optimize it,” Theraulaz said.
Ultimately, the successful adaptation of natural climate control methods to human structures means saving energy and reducing carbon emissions. But it also means creating buildings that are more in tune with the Earth’s natural rhythms. It is, indeed, beyond biomimicry.
“I think we are just at the beginning of an interesting story,” Theraulaz said.
Mark Wolverton is a science writer living near Philadelphia.
The story begins in 1992, when Zimbabwean architect Mick Pearce received a commission to build Eastgate Centre, a two-building office complex and shopping mall, in the country’s capital city of Harare. Pearce, however, wanted to do more than just build a new building. He wanted to eliminate the huge heating and cooling plants a 340,000 square feet development typically needed.
Pearce already had a solution in mind. He began to formulate it while watching BBC’s natural history series, Life, on TV one evening.
“I saw David Attenborough climbing inside the chimney of a termite nest in Nigeria,” he said, and he realized that evolution had already solved the problem.
Pearce had grown up in Zimbabwe and was familiar with such chimneys. They rose six to 30 feet above termite nests and were strong enough for elephants to use to scratch their flanks.
Below the chimneys, the termites farmed fungi for food. While the termites relied on soil’s thermal storage capacity to help keep temperatures stable, Pearce realized that the termites also had to breathe.
It was an elegant design. The hot air generated by the nest and its fungus farm, which had higher concentrations of carbon dioxide and methane, exited through the chimney through convention. As the hot air left, it pulled in fresh air from the surface through moist foraging tunnels, which added water vapor to the stream. The chimney, warmed by the sun, heated the air exiting through it, adding an extra push to the convection cycle.
“The termite nest was warmer than the outside, particularly at night,” Pearce said in an interview. “You get air rising out the chimney, and that pulls air in from the holes they go out to forage. That’s how they breathe.”
If termites could do it, Pearce thought, why not humans?
Eastgate
Pearce had one thing going for him: Although Zimbabwe is semitropical, Harare’s 4800 ft. elevation keeps it cool and provides an average temperature swing of about 10 degrees Celsius each day. Pearce figured he could pull in night air to cool concrete and masonry structures. They would act like the soil in a termite nest to store cooling. He could then chill incoming air by running it over those structures during the day.
In the building that Pearce and multidisciplinary engineering firm Arup Group designed, each floor had air ducts running underneath it. These ducts contained concrete blocks with protruding teeth, whose high surface area enabled them to cool down and heat up efficiently.
Banks of fans drove the cool air through the shopping area and seven floors of offices. As the air warmed, it rose and exited through a central atrium connecting the two towers and 48 brick chimneys that ran along their roofs.
Pearce’s designed also used several other tricks inspired by nature. Office windows, for example, are shaded by overhangs and plants to keep sunlight from heating the building too quickly. The exterior features jagged gabbles and facades that act like cactus spikes, whose high surface areas disperse heat rapidly at night.
On many levels, worked spectacularly well. Eastgate cost 10 percent less to build than a similarly sized building with air conditioning. The building also used 35 percent energy when comparable buildings in Harare and saved $3.5 million in energy costs during its first five years.
Further Reading: Storing Heat from Nuclear Power Plants Could Improve Output
Of course, office temperatures are not as tightly controlled as conventional buildings. Most of the time, they hover in the mid-70s degrees Fahrenheit range, but they may reach 80-82 degrees at the end of the day for several weeks each year. This led Pearce and Ove Arup to improve the control system.
“The trick is to coordinate the weather outside with the controlled internal environment, taking into account lag times,” he said. “It’s like tuning an instrument, like an organ in a church.”
Pearce was a pioneer of biomimicry, which draws inspiration from the natural world for human design. He went on to apply his approach to a more efficient office block in Melbourne, Australia, and build a tower and dining hall in China.
“I was convinced that by looking at animal architecture, there are important clues for an architecture which inspires the design team to make buildings that follow natural processes, cycles, and systems,” he said.
Yet despite his successes, Pearce did not learn everything termites had to teach him.
Beyond Biomimicry
Since Eastgate, scientists have learned much more about how mound-building termites (and other animals) work their architectural magic. This has led to a new approach that physiologist J. Scott Turner and architect Rupert C. Soar call “beyond biomimicry.” They want to create “buildings that are not simply inspired by life,” they wrote in a 2008 paper, “but that are, in a sense, as alive as their inhabitants.”
New research techniques that can reveal the unseen intricacies of natural structures makes this goal possible.
One example involves the use of 3D X-ray tomographic imaging. Kamaljit Singh of the Department of Earth Science at Imperial College London and his colleagues used the technique to image sections of termite nests from Senegal and Guinea. This provided unprecedented details of the nest wall microstructure.
While both nests were built by the same species, Trinervitermes geminatus, they used very different construction materials, depending on the local environment. Yet both sets of walls showed similar—and surprising—features.
All the walls contained an intricate network of micropores, with smaller pores deep inside the nest transitioning to larger pores near on outer walls. This network facilitates the exchange of carbon dioxide with the outside and drain rainwater from the nest by capillary action.
When the researchers used their 3D tomographic images to simulate these processes, they found they operated much like gaseous diffusion within human lungs.
The work demonstrates that Pearce’s Eastgate design, as successful as it is, got it wrong.
You May Also Like: Origami Robots Move Like Ants and Work Like Ants
“Eastgate Centre was built on a wrong conception of how termites regulate the temperature of their nests,” Singh’s co-author, Guy Theraulaz of the University of Toulouse, said. “Still, Eastgate works.”
“It is appropriate to speak of [Eastgate] as ‘termite-inspired’ design,” Turner, a biologist at the SUNY College of Environmental Science and Forestry, added. “The architect, Mick Pearce, drew more on inspiration rather than knowledge of how these mounds worked.
“In the end, he created a successful building design because he approached the engineering challenge—a building in semi-tropical climate without an air conditioning plant—in an imaginative way.
“What made this design successful was several ‘termite-inspired’ ideas, like tall stacks, porous walls, and a large central atrium that could draw air passively through the building. What is often not mentioned is the clever use of large heat storage blocks that could damp temperatures through the hot day, while using high-powered fans to ‘empty’ those heat stores during the cool night. Termites do not have the latter. Termites do not use this to maintain temperature.”
So if the Eastgate model isn’t quite right, what is?
Scientists are discovering that termite heating and cooling is far more subtle and complex—especially because different termite species use different techniques, depending on their individual environments and traits.
The species that inspired Mick Pearce and his Eastgate Centre design farms fungus, and the termites must remove the carbon dioxide the fungus generates so they do not suffocate. That problem is solved by the large chimney structures.
Other species, such as the one studied by Singh, build closed nests with no ventilation to the outside world. Instead of chimneys, they use the micropore structure to let the nest “breathe” like a lung.
“Breathing” Mounds
While it’s been known for some time that termites had developed some sort of mechanisms for controlling the internal environment of their nests, researchers disagreed on the details.
Termites obviously used convective process to circulate heat and air, but what drove it? Did the wind create differences in air pressure between the nest interior and exterior, inducing a Venturi flow of air? Was it an internal metabolic process?
It was challenging to pin down the mechanism without complete or direct measurements. This made it necessary to infer interior temperatures, air flow rates, CO2 concentrations, and other variables. The differences between termite species made it all more complicated.
Harvard physicist and mathematician L. Mahadevan and his colleagues, including Turner, Soar, and Samuel Ocko, have tried to cut through the confusion to find some broader principles that held across various termite species.
First, they needed better data. Mahadevan put together an interdisciplinary team, which developed a custom sensor to measure airflow within the mounds themselves. In 2017, they conducted more than one hundred measurements daily in 30 mounds of the African species Macrotermes michaelseni to get a 24-hour picture. They also measured external winds as well as CO2 and temperature in various parts of the mounds.
The team then compared the results with similar measurements they made on Asian termites in 2015. Mahadevan, Ocko, and Alexander Heyde put it all together in a mathematical model that they published in 2019.
The lung analogy turns out to be even more apt than previously thought. The mounds “breathe” both out and in, as the temperatures vary over the course of a 24-hour period.
“One needs to have variations in diurnal temperatures that can drive circulation because of temperature gradients that switch between day and night,” Mahadevan explains.
Recognizing the role of temperature was hardly a new insight, he said. “The innovation was in thinking about the problem differently—by recognizing that diurnal variations can drive flows that ventilate the mound.”
As the heating sunlight moves across the surface of the mound in the course of the day, different parts warm up and then cool. This shifts the temperature gradients inside the mound, which drives the internal airflow. Instead of relying on wind blowing over the mound, termites manage airflow within the nest with something even simpler and more predictable: sunlight. In a real sense, these insects, or at least their climate control, are solar powered.
According to Mahadevan, these daily temperature oscillations drive convective flow, which reverses twice a day and thus serves to ventilate the mound. The study showed that neither wind (of which there was little to be found locally) nor termite metabolism played a role in ventilation, contrary to prior hypotheses.
The same principles should hold across the termite universe, for the fungus-farming chimney builders as well as their less agriculturally inclined, closed-mound living relatives.
“Because daily oscillations in radiative heating are a fact of life on our planet, this mechanism is likely to be generic across different species of mound-building termites,” Mahadevan wrote in his 2017 study. In fact, the researchers speculated that such other animals might take advantage of solar heating to control their ventilation as well.
Often, termites actively manage this process, blocking and opening new tunnels to modify airflow.
That works for termites, but what about human beings? Mahadevan believes there’s no reason that architects could not apply the same principles to their buildings.
Recommended for You: Bots and Bugs in Harmony
“This ought to work in buildings once we recognize that differential heating of the center and the periphery suffice to drive flow,” he said.
Unfortunately, what ought to work in theory does not always pan out in the real world.
Instead, “the mechanism that you find at the scale of the termites has to be adapted,” Theraulaz said. “So we have to discover new principles to design the architecture to get the same kind of properties. When we understand what happens at the microscale, we can build models and try to see what kind of architecture we can build.”
New technologies, such as Mahadevan’s sensors and simulations, promise that easier.
“We now have a set of techniques by which we are able to analyze nest architectures,” Theraulaz said. “In the past 10 years, we have scanned more than one hundred termite nests, so we have a huge basis [for analyzing their] architecture.
“We can apply the same [simulation] techniques to achieve a broader understanding of how these structures play a role in thermoregulation. For the moment, we don’t have a clear idea. Perhaps there are multiple ways to achieve the same kind of thing. It’s something we are beginning to explore.
“The most interesting thing is that we can apply the same tools we use to analyze the nest architecture to human architecture, to analyze the diffusion of gases, how temperature behaves inside. And by simulation we can try to find the parameters in the architecture that will optimize it,” Theraulaz said.
Ultimately, the successful adaptation of natural climate control methods to human structures means saving energy and reducing carbon emissions. But it also means creating buildings that are more in tune with the Earth’s natural rhythms. It is, indeed, beyond biomimicry.
“I think we are just at the beginning of an interesting story,” Theraulaz said.
Mark Wolverton is a science writer living near Philadelphia.