Battery-Based Desalination Made Possible
Battery-Based Desalination Made Possible
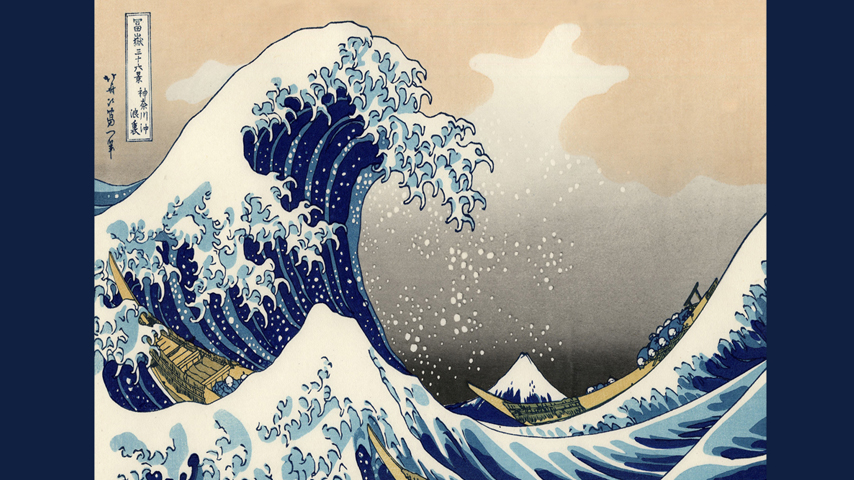
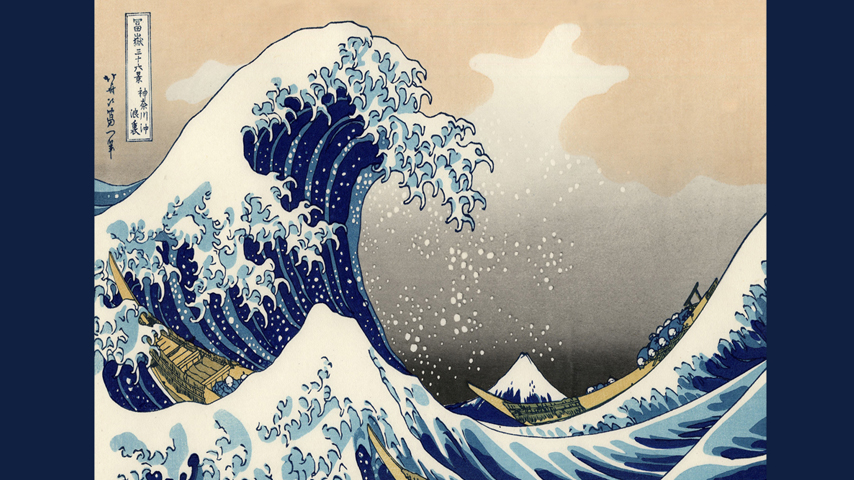
The phenomenon that makes lithium-ion batteries possible also makes the battery a potential tool for desalination, with just a little laser-made channeling.
Moving parts are not the sort of thing one associates with lithium-ion batteries, but at the sub-molecular level, there’s the potential for motion. Run a lithium-ion or sodium-ion battery at a high-enough current and salt scoots away from one electrode and collects on the other. Since the salt depletion effect leads to a drop in capacity, it’s engineered out of a battery design, usually by making the electrodes thin. But anything that moves salt has the potential for an application arguably more important than chemically stored energy: desalination.
“I started thinking, well, how can we harness this effect,” said Kyle Smith, a professor of mechanical science and engineering at the University of Illinois Urbana-Champaign.
Smith found the answer, in part, in Prussian blue, a dye whose chemical analogues researchers have used for years to coat electrodes so that they can better capture and release sodium ions.
“In principle, if a battery uses some kind of material inside it to absorb sodium, there should be some means of leveraging that mechanism to do desalination,” Smith said.
Similar Reading: Optimized Electrodialysis for Wastewater
For years Smith has been working with the dye to create a desalinating battery. Now, after many small-scale successes, he has come upon a technique that removes enough salt from seawater to make it drinkable.
Back in 2016, Smith predicted that “sodium intercalation materials” could desalinate seawater. The next year, he and his colleagues set out to take advantage of a Prussian blue analogue that had the ability to absorb and release atoms of alkali metals such as sodium, potassium, and cesium. They came up with a symmetric cell with a membrane for blocking chlorine transmission. When applying electricity, the membrane generated a stream of desalinated water on one side and a stream of concentrated salt water on the other. It was effective, but only to the tune of half a millimolar concentration of salt from a 25-millimolar saline solution.
As a point of comparison, seawater runs between 30-35 grams of salt per liter of water, or a 500-600 millimolar concentration. In other words, from a not-very-salty brackish water, the researchers extracted a pittance.
Become A Member: How to Join ASME
The primary problem was that the flow channels went behind the electrodes. A low level of electronic conductivity and ionic conductivity also hampered salt removal. Those insights prompted Smith and his team to look for additives that would increase the ionic conductivity of the electrodes. And, to bump up the salt removal, they also forced the fluid to flow through the porous electrodes. That brought the amount removed up to 25 millimoles of salt, 50 times more than their previous attempts. To avoid hotspots of salinity, the team then recirculated the electrolyte at a high rate, bringing the extracted salt up to an 80-millimolar concentration.
While it was a proof of concept, the lab results were a long way from the 500-millimolar salt concentration of seawater. It also took a tremendous amount of energy to pump the water through the tight micron-sized pores of the electrodes to extract that pinch of salt.
“Clearly, the right way to deal with this issue of flow through the porous electrode material was our next step and point of focus,” Smith said. “And the way that we dealt with that was by patterning microfluidic channels into these electrodes using a laser.”
They borrowed the concept of interlocked flow channels from fuel cells and flow batteries. But the channels differed in two essential ways: They were 10 times smaller, and the channels were engraved directly into the electrodes, unlike the plate that the electrodes are up against in a fuel cell.
More on Desalination: Using Solar Power, Forward Osmosis to Desalinate Salt Water
The team also discovered that soaking the electrodes before creating the channels improved their efficiency and minimized degradation of the Prussian blue analogue. The resultant “embedded flow fields,” as they call them, increased the hydraulic permeability by two orders of magnitude. That allowed them to scale up to a bigger cell and to slash the energy needed to pump the electrolyte.
“For the first time, we demonstrated with a flow cell seawater levels of salt removal,” Smith said.
The potential for such a device to provide drinking water from the sea with simplicity and efficiency that beats reverse osmosis is huge. But there’s still work to be done. For one thing, the water they tested the battery on wasn’t actual seawater but a solution with sodium chloride at a similar concentration.
The next step is to further develop the system so that it can also remove other varied elements found in the ocean. And they want to increase efficiency. But Smith and his team have shown how the world could soon have affordable drinkable water wherever there’s ocean.
Michael Abrams is a technology writer in Westfield, N.J.