Slime Fighters: Shear Stress Impacts on Biofilm Activity
Slime Fighters: Shear Stress Impacts on Biofilm Activity
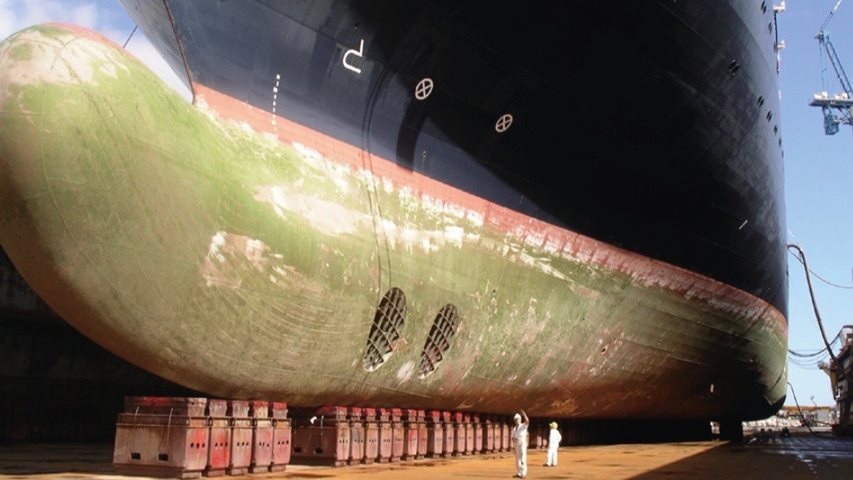
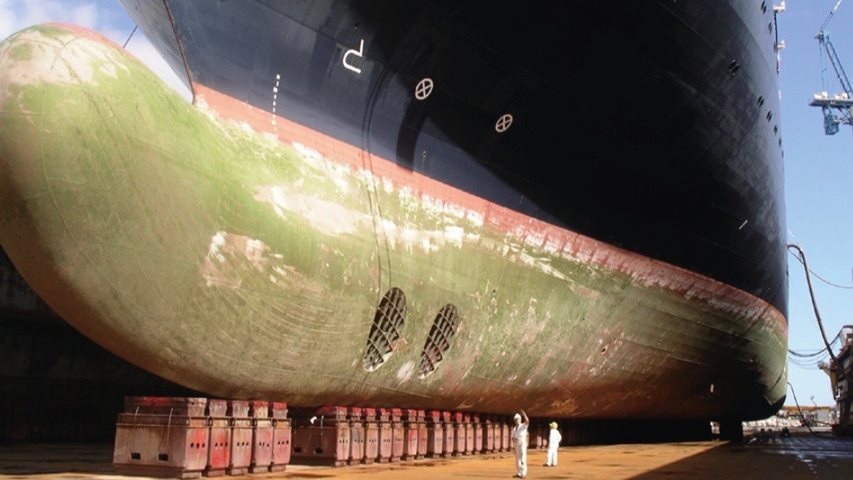
A new model determines the speed and extent of biofilm growth in response to shear stress, better enabling ship operators to schedule hull cleanings, thereby reducing fuel consumption.
Biofilms are a common form of living matter and play an increasingly important role in technology, industry, and health. Although it is well-known that biofilm growth and morphology are highly dependent on shear stress in a flow environment, the precise role of fluid friction has yet to be fully determined.
Biofilm growth (fouling) on the hulls of ships is a major problem. It begins with micro-colonies of bacteria that adhere to a surface and form base structures from which string-like streamers begin to grow. The base structures and streamers combine to form surface imperfections that create friction between the hull and the water. These imperfections build up at different rates, depending on the level of stress induced by water flowing against the hull.
“Slime fouling adds significantly to fluid friction at sea—requiring an increase in a vessel’s shaft power by as much as 18 percent,” said Cornelius Wittig, a doctoral student at the KTH Royal Institute of Technology in Sweden. “A ship’s hull covered with a thin biofilm may experience up to an 80 percent increase in fuel consumption, leading to more emissions and, eventually, higher consumer prices.”
To better understand these negative impacts, Wittig developed a model for estimating the speed and extent of biofilm growth, which can enable ship operators to schedule hull cleaning at more-optimal intervals, which would ultimately save on fuel consumption.
Biofilm analytics
Wittig and his research associates designed an experiment to analyze the influence of wall-shear stress on the growth of Bacillus subtilis biofilm in rectangular channels.
“Biofilms were grown in 12 channels simultaneously, at different shear stresses,” Wittig said. “In this way, we have reduced variations in growth that may be caused by disturbances in the external environment. Using optical coherence tomography, we obtained full volumetric scans of the biofilms every 12 hours for seven days, without disturbing the samples.”
The data was also used to study biofilm morphology, streamer formation, influence of wall shear on biofilm morphology, changes in the substratum coverage, and mechanisms of friction-limited growth.
Discover the Benefits of ASME Membership
By observing the three-dimensional distribution of biofilm over seven days, the team discovered that, “although the shape, size, and distribution of these microcolonies depend on the imposed shear stress, the same structural features appear consistently for all shear stress values,” Wittig explained. “The formation of streamers occurs after the development of a base structure, suggesting that the latter induces a secondary flow that triggers streamer formation.”
The researchers also observed that the biofilm volume grows approximately linearly over seven days for all shear stress values, with a growth rate inversely proportional to the wall shear stress. Using this experimental data, the team developed a scaling model, providing insight into the mechanisms by which friction limits biofilm growth.
Next steps
“This study offers a first-ever explanation of how shear stress interacts with biofilms over time to foster slime fouling on surfaces such as the hull of a ship,” Wittig said. “More research is needed in more realistic conditions before the model can be used in shipping. The evidence shows that shear stress can be roughly estimated by just knowing the speed of a ship. From a ship operator’s perspective, it would be useful to know if the ship should be cleaned next month or next year, so an estimate is sufficient to increase the accuracy of their decision-making.”
The experiment focused on the simplest possible geometry—a channel flow over a flat wall. In the next few years, “we aim to investigate the interaction of biofilms with complex—structured and/or liquid-infused—surfaces,” he added.
Wittig hopes his research will lead to improved models for predicting the early stages of biofouling—possibly in other industries where biofilm is a problem. “For example,” said Wittig, “dependence of biofilm accumulation on wall shear stress might be useful in the design of medical implants, where biofilm-related infections are a common failure mode.”
Mark Crawford is a technology writer in Corrales, N.M.